Resting Eggs of Fairy Shrimp
Introduction to Hatching of Fairy Shrimp
Is Drying Necessary for Fairy Shrimp to Hatch?
How Long Does Fairy Shrimp Hatching Last?
Temperature Effects on Fairy Shrimp Hatching
Total Dissolved Solids Effects on Hatching
Oxygen Concentration Effects on Hatching
Carbon Dioxide Concentration Effects on Fairy Shrimp Hatching
Light Effects on Fairy Shrimp Hatching
Other Controls on Fairy Shrimp Hatching
Randomization of Fairy Shrimp Hatching
Resting Eggs of Fairy Shrimp
Branchiopod resting eggs are commonly referred to as cysts and less commonly as diapausing eggs (Fryer, 1996, Bohonak and Whiteman, 1999) or oviparous eggs (Wurtsbaugh and Gliwicz, 2001). I don’t use those terms. I routinely omit the term “resting” when referring to resting eggs. Eggs not in water are necessarily resting eggs.
Eggs in water may or may not be resting eggs. Authors have used various terms for fairy shrimp eggs that are not resting eggs. Eggs in the ovisac that do not have thick shells have been referred to as thin-shelled (Pennak, 1978, p. 334), non-shelled (Belk, 1982), live larvae (Nambu and others, 2004), live birth (Jones and Stokes Associates, 1993b), and ovoviviparous (Wurtsbaugh and Gliwicz, 2001). Artemia that live in permanent water bodies commonly, and perhaps always, produce such eggs in addition to resting eggs. I refer to continuously wet eggs that hatch before or within a few days of release from the ovisac as live eggs even though this definition precludes identifying live eggs until after they hatch. It may be that live eggs do not have a stiff outer shell made with secretions of the mother’s shell gland. Resting eggs do have the outer shell. However, Brown and Carpelan (1971) didn’t mention any difference between eggs that hatched in the experimental water soon after release by the mother and eggs that hatched after drying.
Resting egg diameters range from about 0.12 mm (0.005″) to about 0.55 mm (0.02″) and are loosely related to adult size. They are most commonly 0.200-0.400 mm (0.008-0.016″) (Hill and Shepard, 1997). Artemia franciscana, a small species, has some of the smallest eggs and Branchinecta gigas, the largest species, the largest. Variation within a species is commonly more than 0.10 mm (0.004″) when more than 20 eggs are measured (Hill and Shepard, 1997). Egg sizes for a single species may differ by geographic location. Shantz (1905) measured diameters of 0.162-0.195 mm (0.0064-0.0077″) for 4 eggs of Branchinecta lindahli collected near Laramie, Wyoming, whereas Hill and Shepard (1997) reported diameters of 0.19-0.32 mm (0.0075-0.013″) for 89 Branchinecta lindahli eggs from California. Shantz (1905) measured diameters of 0.308-0.373 mm (0.012-0.015″) for 25 Branchinecta coloradensis eggs from near Pikes Peak, Colorado. 37 Branchinecta coloradensis eggs from California had diameters of 0.23-0.38 mm (0.0091-0.015″) (Hill and Shepard, 1997) [as interpolated from graph by me]. Except for the smallest fairy shrimp eggs, they are large enough to be seen in an ovisac, particularly if they are a different color than the water. Once they leave the ovisac, it takes considerable effort to find eggs in, or separate them from, water or soil.
For comparison, clam shrimp (Branchiopoda: Conchostraca or orders Spinicaudata, Laevicaudata, Cyclestherida) eggs are less than 0.200 mm (Meyer-Milne and others, 2021) or about 0.200 mm (0.008″) for Spinicaudata (Rivas and others, 2018) in diameter and tadpole shrimp (Branchiopoda: Notostraca) eggs are generally 0.400 mm (0.016″) or greater in diameter (Rivas and others, 2018; Meyer-Milne and others, 2021).
Fairy shrimp resting eggs are typically spherical. Meyer-Milne and others (2021) considered the cylindrical eggs they found as being from clam shrimp (Branchiopoda: order Spinicaudata). They also cited a reference partially titled “tetrahedral eggs in the Streptocephalidae”, which is a fairy shrimp family. The surfaces of resting eggs are extraordinarily varied. They may be relatively smooth, bumpy, pocked marked, dimpled like a golf ball, crumpled like a piece of paper, swathed by sharp or rounded ridges in polygonal or irregular patterns, or spiky (my descriptions of the photomicrographs in Hill and Shepard, 1997). In some cases, the eggs are sufficiently detailed and distinct for the species to be identified (Hill and Shepard, 1997) but Zarattini and others (2013) suggested otherwise. Is this evolution run amuck or is there some practical reason why eggs of different species should have such different surfaces?
If egg shapes affect dispersal, then more favorably shaped eggs could be more widely dispersed. Egg collections over the Northern Cape Province of South Africa demonstrated that “smooth”, “nodulous”, and “polygonal” eggs had wider distributions than “crumpled”, “pitted”, or “grooved” eggs (Meyer-Milne and others, 2021). “Smooth” and “polygonal” eggs were the most common. Statistically significant differences in the distribution and frequency of occurrence of the eggs collected may not have been caused by egg morphology, however. Meyer-Milne and others (2021) pointed out that the large, smooth eggs were probably of the tadpole shrimp genus Triops (Branchiopoda: order Notostraca) and the medium size, polygonal eggs were probably of the fairy shrimp genus Streptocephalus. Are the streptocephalids widely distributed because the polygonal eggs are great at dispersal or are the polygonal eggs widely distributed because the streptocephalids are great at colonizing ponds in the Northern Cape Province? And what of the poorly distributed pitted eggs? Why hasn’t evolution eliminated them if dispersal drives evolution and they aren’t as easily dispersed?
Resting Eggs of Fairy Shrimp and Their Hatching – top
Fairy shrimp eggs have different colors, such as “yellow, orange, or light brown” (Johansen, 1921, p. 23). Eggs can also be very pale or white or green (my observations). The key difference may be that eggs with shells are darker than eggs without. For example, Moore (1963) mentioned “[w]hite, unshelled eggs in lateral pouches of oviducts”. It may also be that eggs with shells get darker as they age. Moore (1963) also noted “[e]ggs confined to median ovisac, light or dark brown in color depending upon the extent of shell deposition.” A serendipitous photograph illustrates the color change in the eggs of a single female (Branchinecta coloradensis) in Bivouac Lake (Wind River Mountains). There are 2 rows of white, unshelled eggs in the oviducts at the headward end of the abdomen and a rearward row of presumably shelled, yellowish-brown eggs in the ovisac. The same thing can be seen in the eggs of a Branchinecta paludosa female in “Coyote Lake” (Antelope Hills). However, another female in Bivouac Lake has only white eggs in its ovisac. Their color suggests they are not shelled but their presence in the ovisac suggests they should be shelled. Were the shell gland secretions delayed for these eggs?
Do they sink or do they float? The eggs of Branchinecta mackini sink (Brown and Carpelan, 1971). Johansen (1921, p. 23) noted that fairy shrimp eggs (probably Branchinecta paludosa and Artemiopsis stefanssoni) in Canada and Alaska float. In the high-TDS waters of “Mono Lake” (80,000-100,000 mg/L), eggs of Artemia monica nonetheless sink (Jones & Stokes Associates, 1993b, p. J-2). In the high-TDS waters of “Great Salt Lake”, some eggs of Artemia franciscana float. As explained by Lavens and Sorgeloos (2000), “Collection of Artemia [cysts] from the lake’s surface instead of from the shore combined with airplane spotting of the cyst streaks (Fig. 1) resulted in a tenfold increase in yield.” Other work indicates the percentage of eggs that float in “Great Salt Lake” changes with TDS from about 20% at 125,000 mg/L to about 80% at 175,000 mg/L (Marden and others, 2012). Artemia parthenogenetica eggs in the high-TDS Odiel salt pans of Spain float. Egg concentrations were “often observed at the downwind edge of salt pans” (Sanchez and others, 2007). In the Aral Sea, where TDS is generally 70,000-110,000 mg/L, 11-17% of Artemia parthenogenetica eggs float (Marden and others, 2012).
The future of eggs that sink would vary depending on whether they were released early in a pond’s wet phase and settled near the periphery or were released later and settled near the center. Although Hildrew (1985) didn’t count eggs, he found that 6 times more fairy shrimp hatched from soil cores collected 3-6 m (10-20′) from the pond center than from closer than 3 m to the center. This is an interesting result as eggs near the periphery of a pond would only get wet when the water level again reaches that point. Eggs near the center of a pond could be induced to hatch in the shortest duration ponds. Such ponds might not last long enough for fairy shrimp to mature. On the other hand, eggs on the periphery could miss out on hatching for a long time.
3 species of large branchiopods in Siangtian Pond, Taiwan, had different egg distribution patterns that may reflect different life cycles and egg deposition preferences (Wang and others, 2014). The eggs of the fairy shrimp Branchinella kugenumaensis were found concentrated in the 3 deepest parts of the 60 m by 75 m pond after it dried up. This could be because sexually mature adults were limited to the deeper parts of the pond. The B. kugenumaensis population produces its first eggs 9-12 days after inundation but ponds typically last only 11-17 days (Wang and others, 2014). However, the distribution of the eggs of the clam shrimp Lynceus biformis contradicts this explanation. It takes about 14 days for L. biformis to mature and its eggs were widely distributed (Wang and others, 2014). That contradiction opens up the possibility that B. kugenumaensis females can ascertain the deepest parts of the pond and preferentially release their eggs there. The inhomogeneous distribution of the eggs of the clam shrimp Eulimnadia braueriana also suggests a preference. As it matures in 7-9 days, females are unlikely to be physically limited in where they can release eggs. E. braueriana eggs are widely distributed but above normal densities along a path that has little vegetation when submerged could be due to female preference (Wang and others, 2014).
Interpretation of the data for Siangtian Pond are complicated by the third dimension, depth or time. The sediment samples from which the eggs were extracted were 5 cm deep and probably included eggs deposited over several years. The third dimension is not completely unknown thanks to the 9-year records for Siangtian Pond (Wang and others, 2014). There were relatively long-lived ponds with durations of 24 days in 2013 and 32 days in 2008. All 3 branchiopods matured more than 10 days before the final desiccation of the pond in those years. Assuming that the numbers of eggs released during a pond episode increase with the life spans of mature adults, most of the eggs collected could have been from 2008 and 2013 with lesser contributions from ponds of 17 days durations or less in 2013 (1 pond), 2012 (1 pond), 2007 (3 ponds), 2006 (1 pond), 2005 (3 ponds), 2005 (4 ponds), and 2004 (1 pond). There was also a pond in 2004 that lasted 50 days (Wang and others, 2014). If most of the eggs were from years with long-lived ponds, the observed egg distributions of the 3 species could reflect egg-release preferences, barring differential effects of wind and vegetation due to the strikingly different egg morphologies.
Resting Eggs of Fairy Shrimp and Their Hatching – top
Eggs that float face a risky future. They could be carried to the shore by wave action or blown into the air by wind rather than being deposited on the former pond bottom upon evaporation. In permanent water bodies, floating eggs would almost certainly end up on the shoreline (see the Lavens and Sorgeloos, 2000, quote above). When a pond level drops due to evaporation, stranded floating eggs would be exposed to wind transport and lose their chances to hatch in the home pond. Even if not blown away, they wouldn’t get a chance to hatch unless the lake level again rose to cover them or the waves grabbed them.
Whether floated or sunk, eggs could be carried off in mud stuck to drinking or wallowing mammals and redistributed by bioturbation on a smaller scale by worms and other soil animals. Buried eggs don’t get a chance to hatch. However, they could be brought to the surface again by more drinking, wallowing, or bioturbation.
That not all eggs remain in the home pond is part of the Anostraca plan. If a pond bottom is pulverized to dust, eggs could be carried off by the wind. Eggs in mud could be carried away stuck to the feathers, fur, legs, or exoskeletons of various animals. Fairy shrimp eggs have been found in the feathers of teal (Brochet and others, 2010) and in the fur of mammoths (Rogers and others, 2021). A chance at life would have long odds for such eggs but if they end up in a new pond then that could contribute to the persistence of the species.
Wherever eggs end up, there is a risk of predation. The floating eggs of Artemia franciscana on “Great Salt Lake” are an important food source for northern shovelers and green-winged teal (Vest and Conover, 2011, abstract only). Northern shovelers in particular have lamellae in their bills for straining small food items from the water. On the bottom of a pond, ostracods (Thorp and Covich, 2001, p. 827) and tadpole shrimp (Thorp and Covich, 2001, p. 897) could eat fairy shrimp eggs. Daborn (1977, cited by Thorp and Covich, 2001, p. 898) specifically mentioned tadpole shrimp eating eggs of fairy shrimp and clam shrimp. There are other detritus feeders like snails and worms that might consume resting eggs. Although shells of resting eggs are tough, they might be penetrated by aggressive fungi.
Fairy shrimp eggs are covered by a shell in the ovisac but this is only the start of a process to make the eggs resistant to physical changes that would normally kill. The covered eggs divide a few times and become embryos or, as described by Kaestner (1970, p. 88), “pass through total cleavage and blastoderm formation”. The embryos consist of about 4,000 cells (Dai and others, 2011). 2 cuticles are formed below the shell during 2 successive molts of the embryo (Belk, 1987). The outer cuticle ruptures when the shell does but the inner cuticle remains intact until the emergent nauplius breaks it (Belk, 1987). The shell and the 2 embryonic cuticles are permeable to water for obvious reasons (Belk and Cole, 1975). After some minimal level of development, the embryo becomes dormant and metabolism essentially stops (Belk and Cole, 1975). This state may be referred to as diapause or cryptobiosis. The fact that the egg has become an embryo although dormant and still covered by a shell may be why some people use the term cyst. The term cyst refers to a variety of sac-like biological features, including the one in my nose that hurt when I flew in an airplane.
To demonstrate that the metabolism of resting eggs really does stop, Clegg (1997) subjected hydrated resting eggs of Artemia franciscana to a continuous absence of oxygen using nitrogen gas for up to 4 years. Concentrations of the eggs’ energy sources, trehalose, glycogen, and glycerol, did not change over 4 to 48 months of anoxia. When transferred to aerobic seawater, the eggs subjected to anoxia hatched at rates decreasing from about 90% after 1 year of anoxia to about 60% after 4 years of anoxia (Clegg, 1997). Until hydrated, dried eggs use essentially no energy.
Once the embryo switches off, the resting egg becomes a time capsule that survives in the face of extreme environmental assaults. This is an obvious adaptation to ephemeral ponds that dry up over the course of a season and may not refill again for years. Fairy shrimp eggs are tougher than that though. Maybe their toughness is the legacy of past environmental catastrophes that the order has experienced, such as the end-Cretaceous extinction 66 million years ago and the end-Permian extinction 252 million years ago.
Fairy shrimp eggs survive pond desiccation and exposure to dry soil or an atmosphere with low humidity. However, there is a limit. Moore (1967) found that after storage at 0% relative humidity for 60-90 days, only 5 of 400 Streptocephalus seali eggs hatched (cited in Belk and Cole, 1975). But 5 did hatch!
The shell plays an important role in protecting the egg from TDS shocks (increase or decrease), heat shocks (hot or cold), degradation by methanol, and ultra-violet light exposure. It is permeable to carbon dioxide but not to amino acids, glycerol, or glucose (MacRae, 2016). In experiments with Artemia parthenogenetica, genetic manipulations to produce shells lacking critical peptides resulted in nonviable eggs (Dai and others, 2011). In the worst case shell treatment, eggs shrank dramatically in high-TDS water within 4 hours and burst in deionized water within 16 hours, succumbed to 50 C for 5 min or -30 C for 3 days, died in 0.5 molar methanol, and suffered fatal damage when exposed to 310 nanometer light at 3.6 joules per square centimeter for 30 minutes (Dai and others, 2011). None of such treated eggs hatched after exposure to any one of the environmental stresses. Non-treated control eggs hatched at rates greater than about 60% after each of the environmental stresses (Dai and others, 2011). Further, Pennak (1978, p. 335) found that resting eggs survived -190 C for 24 hours and 81 C for one hour. The shell is critical for resting egg survival.
Resting Eggs of Fairy Shrimp and Their Hatching – top
The embryo within the shell contains key compounds which augment the protections provided by the shell and protect it from various other environmental stresses. Trehalose is a sugar that makes up about 15% of a resting egg by dry weight. It preserves the structures of membranes and prevents protein denaturation without requiring energy (MacRae, 2016), a necessary requirement for an egg without metabolism.
The embryo also contains heat-shock proteins, such as p26, which help prevent denaturation of proteins upon heating. Both p26 and the protein artemin improve tolerance of drying and freezing. p26 and artemin are not present in nauplii. That p26 is not found in live eggs which develop directly into nauplii confirms it as a specialization of resting eggs. Fairy shrimp embryos also contain late-embryogenesis-abundant (“LEA”) proteins which help stabilize membranes, inhibit protein aggregation, increase tolerance to drying and freezing, and preserve the integrity of mitochondria (MacRae, 2016). Although some of the biochemical processes which contribute to the resilience of fairy shrimp eggs have been identified, a great deal remains unknown.
Trehalose plays another critical role when metabolism resumes during hatching. It is the primary source of energy. It is converted to glycogen and glycerol by the enzyme trehalase (MacRae, 2016). Although abundant in the embryo, it is essentially absent from newly hatched nauplii. When a resting egg is inundated, the conversion of trehalose to glycerol is stimulated (Clegg, 1964). As glycerol increases in the embryo, water is pulled into the interior of the shell in response to the chemical gradient. Clegg (1964) calculated that the increase in pressure within the egg resulting from this water influx could result in the rupture of the egg. The sudden and proportional increase in glycerol concentration in water with emerging nauplii further supports the role of glycerol in rupturing the egg shells (Clegg, 1964). Consequently, factors which affect the conversion of trehalose to glycerol may offer a way for fairy shrimp eggs to fine tune their hatching responses.
However they do it, fairy shrimp eggs survive some environmental stresses that aren’t likely to occur on Earth. They survive storage at a pressure that is about one-billionth of atmospheric pressure (“0.000001 mm Hg”) for 6 months (Pennak, 1978, p. 335). Could there be fairy shrimp eggs on meteors or asteroids cruising through the cosmos? There were fairy shrimp eggs on Earth when the asteroid that killed off the dinosaurs hit. Maybe some were carried off on the ejecta.
It’s hard to figure out from abstracts how fairy shrimp eggs do in space. Gaubin and others (1990, abstract only) stated that “space flight resulted in a decrease in developmental capacity of Artemia cysts”. However, a subsequent publication by the same authors about a different space flight stated that Artemia eggs survived 69 months in space with an “unchanged or slightly reduced” survival rate (Planel and others, 1994, abstract only). In the first experiment, Artemia eggs and tobacco seeds were placed inside and outside the spacecraft in “monolayers” or in “bulk”. In the second experiment, everything in monolayers died.
NASA developed a syringe system to combine Artemia franciscana resting eggs, salty water, air, and food for experiments on the Space Shuttle. On Atlantis mission STS-37, 50% of the eggs hatched and most molted 2 or 3 times. The nauplii were not fed and only 10% survived until Atlantis’s touchdown on the 6th day (Spooner and others, 1992). Food was provided for the STS-43 experiments but half of the hatched nauplii were preserved after 2 or 4 days for detailed comparisons to ground-hatched nauplii. An unspecified number survived 8 days until the end of the mission (Spooner and others, 1992). Hatching rates for experiments on STS-50 and STS-54 were similar to those on the ground using the same apparatus (Spooner and others, 1994, abstract only). As all but a few nauplii in the space shuttle experiments appeared to be normal, A. franciscana probably could reach adulthood and produce eggs in space. If nothing else, fairy shrimp eggs could be taken on years-long journeys through space and provide a few weeks of entertainment after being hatched. Sea Monkeys could become Space Monkeys.
For fairy shrimp to survive in California, they have to survive wildfires. And they do. Branchinecta sandiegoensis hatched from soil collected from a pond that was burned over by a 1993 wildfire on Naval Air Station Miramar (Welch and others, 1997). Field surveys during the wet season following the fire also found fairy shrimp in other ponds within the burned area. Egg survival was likely assisted by the light fuel load of the herbaceous vegetation surrounding the fairy shrimp ponds and by the insulating effect of the dry soil (Welch and others, 1997).
Resting Eggs of Fairy Shrimp and Their Hatching – top
In what sounds like bad news, dry fairy shrimp eggs can be crushed by “very small forces of less than 1 newton” and wet eggs are even easier to crush (Hathaway and others, 1996, abstract only). How closely test conditions resemble natural conditions is not evident from the abstract. Hathaway and others (1996, abstract only) implied that fairy shrimp eggs would be susceptible to damage by off-highway vehicle traffic. In spite of that, Vanschoenwinkel and others (2013) found fairy shrimp in 18 ponds along dirt roads in a rural area of Belgium. They also inferred that “military domains in Eastern Europe” have proven to be “particularly suitable” as refuges for fairy shrimp and other large branchiopods due to “natural habitat that was historically set apart, unsealed roads with puddles and wheel tracks and regular disturbance by vehicles”. Stock ponds with fairy shrimp in the western United States prove that egg populations, if not all individual eggs, survive stomping by cows and horses (e.g., Belk, 1977).
There is anecdotal evidence of how long eggs remain viable. Some eggs left in dried pond mud on a laboratory shelf for 15 years hatched (Pennak, 1978, p. 335; also cited by Donald, 1983). Donald (1983) hatched eggs that were 4 years old. Yang and Sun (2023) hatched eggs of Artemia sinica that had been stored by the Artemia Reference Center at Ghent University since 1991. They did not say when their experiments were conducted but it could have been 5-30 years after the eggs were collected. Rode and others (2011) hatched Artemia franciscana eggs collected from “Great Salt Lake” in 1985, 1996, and 2007 and stored at the Artemia Reference Center. The experiments could not have been conducted before 2007 so the 1985 eggs that hatched were at least 22 years old.
Fairy shrimp eggs depend to a large extent on animal vectors for dispersal from one pond to another. If only a few eggs get stuck to an animal in fur, feathers, or dried mud, there is a risk that not enough eggs will hatch in the new pond to create a new generation of eggs that will be large enough to give rise to a sustainable population. Consequently, predators that ingest female fairy shrimp with full ovisacs whole are better dispersal agents from the perspective of the species than animals with a few eggs stuck to their bodies. For this to work, the eggs must survive the relatively acid, enzyme-rich environments of predator digestive tracts.
Birds are probably the principal long-range dispersal agents for fairy shrimp. At least some Artemia eggs recovered from the feces of mallards, shelducks, killdeer, and flamingos hatched in experiments (Figuerola and Green, 2002, Table 1). Van Stappen (1996) also noted the role of flamingos in the dispersal of Artemia. He added that the eggs survived in the digestive tracts of birds “for at least a couple of days”. “Large numbers” of Artemia franciscana and A. parthenogenetica eggs recovered from the feces of redshank and blacktailed godwit migrating through Castro Marim, Portugal, and Cadiz Bay, Spain, were found to be viable (Green and others, 2005, abstract only). Other bird collections from the Odiel marshes, Spain, had Artemia eggs in spring or autumn but not in winter (Sanchez and others, 2007; Frisch and others, 2007). The strong correlations between numbers of eggs and proportions of Artemia adults in the fecal or pellet samples indicated most eggs were derived from egg-bearing females (Sanchez and others, 2007).
About 1 percent of eggs in salamander feces hatched in the field experiments of Bohonak and Whiteman (1999). The low hatching rate may be because not all the eggs completed the transition to diapause before consumption. Earlier work by Whiteman and others (1994) (as cited by Bohonak and Whiteman, 1999) had found an average of more than 70 adult fairy shrimps in the guts of salamanders. Assuming half were females with an average of 18 eggs each (n=42, Bohonak and Whiteman, 1999), salamanders at that locality carried around 600 or so eggs each, on average. The numbers of eggs per defecation for such salamanders could be substantial so even low hatching rates could result in successful colonization.
Resting Eggs of Fairy Shrimp and Their Hatching – top
Beladjal and others (2007a) cited a work by Mathias (1929) that found fairy shrimp eggs remained viable after passage through the digestive tracts of frogs.
Even fish! About 50% of fairy shrimp eggs recovered from the feces of brown trout hatched in an experiment by Beladjal and others (2007a). This study was motivated by the unexpected observation of fingerling trout in ponds with fairy shrimp in the French Pyrenees. It turns out that some small lakes that freeze solid in winter are connected by small streams that fingerling but not adult trout can swim up. After hatching, the fingerlings swim up the small streams to previously frozen, fish-free lakes. By that time, any fairy shrimp have matured and reproduced. If the fingerlings reach a pond with fairy shrimp, they eat them all. The fingerlings could carry the eggs of any females they ate to other lakes they may visit. Any fish that remain in a fairy shrimp pond die when it freezes solid. Next spring, fairy shrimp can hatch and mature before a new batch of fingerlings finds them.
As amazing as fairy shrimp resting eggs are, they are not alone in the animal world. Species which have resting eggs are most common in the class Branchiopoda. This includes Anostraca (fairy shrimp), Notostraca (tadpole shrimp), Spinicaudata (clam shrimp), and the cladoceran orders Anomopoda, Ctenopoda, Onychopoda, and Haplopoda (Hairston and Caceres, 1996). 5 species of Anomopoda have eggs that survive for more than 30 years. Eggs of the notostracan Triops longicaudatus are good for 15 years while 5 of 6 surveyed spinicaudatan species have eggs which last about 5 years. Diapause is associated almost exclusively with “inland waters” (Hairston and Caceres, 1996). Hairston and Caceres (1996) found that of 167 species of 20 crustacean orders from the classes Branchiopoda, Malacostraca, and Maxillopoda, those that have resting eggs which remain viable for more than a year (“duration of diapause”) generally have life spans less than a year. Most crustaceans are marine and don’t need diapausing eggs because that environment is relatively unchanging.
It’s not just crustaceans though. Some rotifers, nematodes, and insects produce diapausing eggs (e.g., Frisch and others, 2007; Rivas and others, 2018).
Resting Eggs of Fairy Shrimp and Their Hatching – top
Introduction to Hatching of Fairy Shrimp
Having eggs that survive everything the environment throws at them is not enough. The eggs have to hatch. Because adapting to predator-poor environments with wide ranges of physical and chemical conditions is a key life strategy of the order Anostraca, fairy shrimp eggs necessarily hatch over wide ranges of physical and chemical conditions. Hatching of fairy shrimp resting eggs has attracted considerable interest. Some of the experimental work is summarized here.
When considering experimental results, it is important to realize that eggs that don’t hatch under certain conditions in a hatching experiment may be dead, may hatch under different conditions, or may hatch under those same conditions in some future inundation.
Resting Eggs of Fairy Shrimp and Their Hatching – top
Is Drying Necessary for Fairy Shrimp to Hatch?
Pennak (1978, p. 335) dismissed the suggestions by some that a period of freezing or drying or both are necessary for the eggs of some species to hatch. If fairy shrimp species or populations progressively adapt to their local environments, though, losing the ability to hatch without drying after many generations seems plausible.
For example, Streptocephalus vitreus from Kenya may require drying. Eggs kept wet as controls for the 112-day duration of experiments never hatched (Hildrew, 1985).
Vanschoenwinkel and others (2010) stated that “[s]ome resting eggs, however, are not responsive to hatching cues and require desiccation, a critical amount of time or a cold shock before they hatch (reviewed in Brendonck and De Meester 2003).” Regarding the genus Branchipodopsis, “[e]ggs typically require a period of desiccation before they can hatch (Vanschoenwinkel unpublished data).”
Although ephemeral water bodies are characteristic habitats of the order, there are many examples of fairy shrimp hatching without drying. The most obvious are in permanent high-TDS lakes. In “Mono Lake”, California, and “Great Salt Lake”, Utah, adults of Artemia monica and A. franciscana, respectively, die off in the winter. The eggs of both populations are released into the water and necessarily hatch without drying. The populations have persisted over more than 150 years of observation. For example, in 1872 Mark Twain wrote of A. monica: “There are no fish in Mono Lake . . . no living thing exists under the surface, except a white feathery sort of worm, one-half an inch long, which looks like a bit of white thread frayed out at the sides” (Mark Twain, 1872, Chapter 38 in “Roughing It”: Reader’s Digest Association, Pleasantville, NY, 1994, p. 170-171).
Artemia urmiana has been documented in “Lake Urmia”, Iran, for at least 1,000 years (Djamali and others, 2010). “Lake Urmia” is very large. Djamali and others (2010) wrote that the water level of “Lake Urmia” varies greatly from year to year but not that it goes dry. Agh and others (2008) called “Lake Urmia” permanent while mentioning that the lake’s area had contracted to 4,000 square kilometers by 2006 due to drought since 1999.
The ability to hatch without drying has been demonstrated for at least a few populations living in ephemeral water bodies. The hatching experiments of Brown and Carpelan (1971) included “ejected eggs” of Branchinecta mackini from “Rabbit Dry Lake” in the Mojave Desert of California. Females with full ovisacs were placed in vials with water at various test concentrations and removed after their eggs had been released into the water. Hatching rates of these “ejected eggs” were then observed for different test concentrations of TDS. Brown and Carpelan (1971) were primarily interested in how hatching rates varied with TDS but they noted that “ejected eggs” hatched easily without a period of drying or undergoing diapause – so easily that they “seem to require some inhibitor to prevent hatching” (p. 53). Brown and Carpelan’s (1971) experiments with “ejected eggs” indicate that even these desert-dwelling, clay-loving fairy shrimp can hatch and live in perennial water bodies. If only they get the chance.
In a field experiment, Bohonak and Whiteman (1999) collected eggs in much the same manner as Brown and Carpelan (1971). Egg-bearing Branchinecta coloradensis females from temporary ponds in Colorado were placed in vials. The vials, with mesh coverings, were transferred to other temporary ponds or to permanent ponds in the same area. The females died in the winter, if not before, and their eggs remained in the vials. Eggs in vials transferred to permanent ponds hatched at an average rate of about 50%, which was not significantly different from the rate for eggs that had dried after being transferred to temporary ponds.
Eggs of Branchipus schaefferi and Streptocephalus torvicornis hatched without drying in the experiments of Beladjal and others (2007b). Hatching rates in the distilled water controls were 56% and 89%, respectively.
In the experiments of Saengphan and others (2005), eggs of Branchinella thailandensis hatched at rates of 75-99% when not dried. These rates were higher than the hatching rates after various periods of drying in 4 of 6 other treatments.
Resting Eggs of Fairy Shrimp and Their Hatching – top
How Long Does Fairy Shrimp Hatching Last?
The hatching process can be divided into 2 steps. The first step is hydration as the egg absorbs water. The shelled embryo’s metabolism is activated when hydration changes the concentration of water within the resting egg from less than 30% by weight to more than 60% by weight (MacRae, 2016). Naturally dried eggs of Branchinecta mackini are conveniently indented. They become spherical after absorbing water so their hydration can be observed under a microscope. Brown and Carpelan (1971) measured the time it takes for half of 100 tested eggs to become round as 88, 117, and 172 minutes in waters with progressively higher TDS concentrations as indicated by specific conductances of 1,000 (about 730 mg/L TDS), 10,000 (6,000 mg/L TDS), and 20,000 micromhos (11,900 mg/L TDS). The water of hydration provides the embryo information about its environment. That, and other factors, control whether or not the embryo breaks diapause and subsequently hatches.
After a resting egg is hydrated, the second step is for the embryo to re-start development. One-half to a few days later, the embryo becomes a nauplius and breaks out of its shell.
There is also something called “prehatching”. In habitats that don’t dry out, Eubranchipus grubii (Rogers, 2013; Siphonophanes grubei in original) eggs kept in water above 15 C for at least 21 days and then cooled to less than 10 C prehatch in the following 42-136 days (Mossin, 1986, abstract only). In prehatching, the hard outer shell is broken but the inner cuticles remain intact. The embryo remains dormant until stimulated to hatch by appropriate environmental conditions. Prehatching can’t be observed in the field. There, the emergence of nauplii would be interpreted as hatching regardless of whether or not there was a prehatching phase.
The hatching period for a population of dried fairy shrimp eggs lasts for 1 to several days.
In Brown and Carpelan’s (1971) hatching experiments, hatching of dried eggs began within 24-36 hours and counting of hatched nauplii stopped at the end of 5 days. In the field, the continued presence of nauplii was used to infer continued hatching. Durations of the field hatching periods ranged from 1 day at relatively high TDS and a rapid rate of TDS increase to 38 days at the lowest observed TDS and rate of TDS increase (Table 3, Brown and Carpelan, 1971). The inverse relation between hatching duration and rate of TDS increase reflects the likelihood that a rapid rate of increase indicates the pond is drying up. The nauplii of eggs that wait too long to hatch may not have time to mature.
Daily observations by Vanschoenwinkel and others (2010) found that nauplii of the genus Branchipodopsis appeared on days 2-8 after initial inundation of 15 rock pools of various sizes in South Africa. Their Figure 2 indicates the bulk of the hatching occurred on days 4 and 5 and almost none on days 1, 2, 7, and 8.
In hatching experiments with Thamnocephalus platyurus eggs, less than 5% hatched on day 1 and the most hatched on day 4 (Murugan and Dumont, 1995). The daily hatching percentage approximates a bell-shaped curve out to day 6.
Chirocephalus ruffoi is a slow hatcher. Its eggs hatched over periods of time from 2 to 14 days after immersion (Mura, 2001). Different experiments exhibited different hatching curves but most hatching across all experiments occurred on days 6 through 9.
Streptocephalus vitreus is a quick hatcher. In experiments with eggs from a pond in Kenya, 24.5% of all the eggs that hatched hatched within the first day, 74.5% on the second day, and 1% on the third day (Hildrew, 1985).
Hatching of non-dried, live eggs is quick in some species. In experiments with Streptocephalus torvicornis, about one quarter of all the eggs that would hatch for that treatment had hatched by the end of day 1 and three quarters by the end of day 3 (visual estimates of graphs for treatments Cd, EPA, and dw in Figure 1 of Beladjal and others, 2007b). There was additional hatching on day 4 and essentially none after day 5. Non-dried eggs of Branchipus schaefferi hatched even quicker. More than 80% had hatched at the end of day 1 and essentially none hatched after day 2 (visual estimates of graphs for treatments St, Cd, and dw in Figure 2 of Beladjal and others, 2007b).
Live eggs are slower to hatch in other species. In Brown and Carpelan’s (1971) experiments with “ejected” live eggs, hatching began after 2 days in low TDS water (1,000 micromhos = 730 mg/L), after 4 days in moderate TDS water (2,600 micromhos = 1,670 mg/L), and after 4 days in high TDS water (10,000 micromhos = 6,000 mg/L). The corresponding hatching observation periods were 5 days, 9 days, and 12 days. Those periods were presumably based on hatching durations. In general, live eggs began hatching later than dried eggs in water with the same TDS and continued hatching for longer (Brown and Carpelan, 1971).
Like other fairy shrimp life characteristics, the beginnings and durations of hatching vary by species, individual, and environmental conditions. To find visible fairy shrimp, the fairy shrimper has to consider how long it takes after inundation before hatching begins and how long it takes before nauplii grow to a visible size. My guess is that in most cases, 1 week after inundation isn’t long enough, 2 weeks would be okay for quickly growing fairy shrimp, and 4 weeks would work for most species but could be too late if the pond is small, shallow, and in an arid environment.
Resting Eggs of Fairy Shrimp and Their Hatching – top
Temperature Effects on Fairy Shrimp Hatching
Just as the temperature effects on growth and life span have been investigated so have the effects on hatching. Belk (1977) determined hatching rates for 9 species of fairy shrimp from Arizona at temperatures of 5 C to 40 C at 5-degree intervals (except 42 C for S. mackini) but showed only graphs of the results. The ranges of temperatures over which the eggs hatched and the temperatures with the highest mean hatching rate are as follows.
- Branchinecta paludosa: range 5-10 C; highest rate at 5 C.
- Eubranchipus bundyi: range 5-10 C; highest rate at 5 C.
- Eubranchipus serratus: range 5-15 C; highest rate at 5 C.
- Branchinecta packardi: range 5-25 C; highest rate at 5 C but hatching at 20 C still more than 50%.
- Branchinecta lindahli: range 5-25 C; highest rate at 5 C but 15 C and 20 C also had rates greater than 80%.
- Thamnocephalus platyurus: range 15-35 C; highest rate at 20 C.
- Streptocephalus dorothae: range 15-35 C; highest rate at 20 C.
- Streptocephalus mackini: range 15-42 C; highest rate at 25 C.
- Thamnocephalus mexicanus: range 20-35 C; highest rate at 25 C.
The first 3 species above clearly have higher hatching rates at low temperatures. B. packardi and B. lindahli are generalists which hatch at high rates in cool water but also hatch at significant rates in warm water. Horne (1967) similarly found that Wyoming populations of B. packardi and B. lindahli hatched at temperatures of 9 C, 12 C, 15 C, and 18 C. In contrast, the last 4 species above didn’t hatch at all below 15 C and all had at least small hatches at 35 C. Deionized water was apparently used in all experiments so hatching rates in real ponds could differ from these results. Notably, the 4 warm-adapted species all had maximum hatching rates less than 80% and mostly less than 65%.
The graph “Fairy Shrimp Hatching Rates vs. Temperatures for Eggs Collected from Iran” indicates that hatching rates for one population of Branchinecta orientalis vary substantially over the range of 12-27 C. Hatching rates were better than 50% over the range 15-24 C and the highest rate was at 21 C. Data in Atashbar and others (2012) gave exactly equal 1st day and 10th day cumulative hatching rates at temperatures of 12 C and 27 C. This implies only 1 day of hatching. The 1st day results for these temperatures are not shown on the graph due to lack of explanation for this puzzling result in the text of the article.
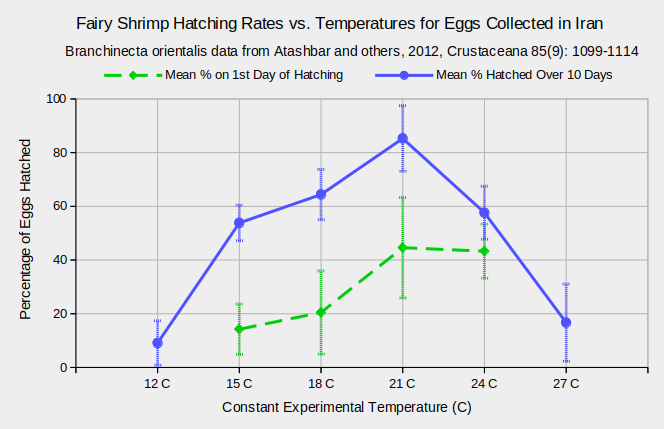
Temperature is probably the major control for hatching of Artemia monica in “Mono Lake”, California, but there is no simple threshold. 3 months in water colder than 5 C has been suggested as a prerequisite for resting eggs to hatch (Dana, 1981, and Thun and Starrett, 1986, as cited by Jones & Stokes Associates, 1993b, p. J-4). Hatching due to temperatures above 5 C would make sense. However, in experiments, some eggs hatched at 2.5 C. It just took them an average of 42 days after the experiment began (Jones & Stokes Associates, 1993b, p. J-4). It was also noted that nauplii that emerge from eggs at temperatures less than 6 C rarely survived (Jones & Stokes Associates, 1993b, p. J-4). In the lake itself, hatching occurs in the spring when surface water temperatures are generally 5-15 C (Jones & Stokes Associates, 1993b, p. J-3).
Eggs from different populations of Branchinecta lindahli in 4 widely separated geographic regions of California responded differently to temperature. Eggs of each population were exposed in the laboratory to the temperature conditions of all 4 locations Rogers (2015b). The hatching rate for each of the 4 populations was always greatest for the temperature conditions of their home ponds. At the extremes, the southernmost population did not hatch at all under the coolest conditions and the northernmost population did not hatch at all under the warmest conditions (Rogers, 2015b). Environmental factors can thus change the responses of eggs from different populations of the same species. In effect, eggs “remember” their parents’ pond conditions.
Resting Eggs of Fairy Shrimp and Their Hatching – top
Total Dissolved Solids Effects on Fairy Shrimp Hatching
First, fairy shrimp eggs don’t need dissolved solids to hatch. Some species hatch in distilled water (e.g., Mura, 2001, Beladjal and others, 2007b). But natural waters, even rain, have various amounts of dissolved solids. Because many aquatic predators are absent from waters with TDS concentrations greater than those of seawater, fairy shrimp hatch in those waters as well. However, no single species of fairy shrimp can hatch, or survive, in waters spanning the range of habitats from, say, 10 to 150,000 mg/L total dissolved solids. The physiology of osmoregulation (on Habitats of Fairy Shrimp page) has limits. Even so, most species can hatch over wide ranges of TDS.
Higher TDS leads to lower experimental hatching rates in Artemia monica in the permanent “Mono Lake”. The exponential equation for the percentage of eggs that don’t hatch as a function of salinity in Table J-1 of Jones & Stokes Associates (1993b) gives hatching rates of 88% at a TDS concentration of 60,000 mg/L, 73% at 100,000 mg/L, and 37% at 140,000 mg/L with a coefficient of determination (r-squared) of 0.68. Hatching rates in the bar graph of Dana and Lenz (1986, Figure 5) look like about 70% at 76,000 mg/L, 50% at 97,000 mg/L, and 20% at 133,000 mg/L. None hatched at 159,000 mg/L. TDS of the lake was about 100,000 mg/L in 1993 (Jones & Stokes Associates, 1993b, p. J-4) and changes “relatively little” due to spring run-off and summer evaporation (Jones & Stokes Associates, 1993b, p. 3E-3). The current population has experienced larger year-to-year changes. TDS was 50,000 mg/L in about 1940 (Herbst, 2014, but source not cited), 100,000 mg/L in about 1980 (Herbst, 2014, but source not cited), 88,000 mg/L in 1983 at 8 m depth (Dana and Lenz, 1986), and back to about 80,000 mg/L in 2002 (He, 2021 but source not cited).
The effects of TDS on hatching are not affected by whether sodium or potassium is the only cation or whether nitrate or chloride is the only anion in some cases. Similar mean hatching rates of non-dried Streptocephalus torvicornis eggs were observed for solutions with the same conductivities (and therefore TDS) that had been prepared with sodium nitrate (56% hatching rate), sodium chloride (54%), potassium nitrate (52%), or potassium chloride (46%) (Beladjal and others, 2007b). Standard deviations for 10 replicates of 100 eggs each were 8% to 18%. These hatching rates were lower than the hatching rate of 89% in the control with distilled water.
The physical reality of ephemeral ponds is that TDS is at a minimum when the ponds first fill and increases progressively as the pond evaporates. In ponds that precipitate a significant mass of soluble minerals upon final evaporation, dissolution of those minerals during the next wet phase results in an initial TDS higher than that of the new water influx. Most of those minerals would be concentrated where the last water of the previous wet phase evaporated and consequently near the deepest parts of the pond. With or without the presence of soluble minerals, the larger the volume of the water influx from melting snow, rain, and overland and channel flow, the lower the initial TDS of the new wet phase and the deeper the starting depth of the pond. The deeper the water, the longer it will take the pond to evaporate, and the longer fairy shrimp will be able to live. This would be useful information for a fairy shrimp egg to know.
Some fairy shrimp eggs do sense and use TDS information. The hatching pattern of dry fairy shrimp eggs of the “Rabbit Dry Lake” population of Branchinecta mackini is strongly related to TDS and its experimental proxy, specific conductance. Using sample sizes of 234-897 eggs, Brown and Carpelan (1971) counted how many of the eggs hatched in waters with specific conductances of 360-20,000 micro-mhos (now micro-Siemens). For reference, 360 micro-mhos corresponds to a TDS of 350 mg/L and 20,000 micro-mhos to 11,900 mg/L based on the relation used by Brown and Carpelan (1971, Fig. 1 and Table 2). Biologists find it easier to measure specific conductance or electrical conductivity as a measure of TDS but fairy shrimp can sense TDS directly as the osmotic pressure differential across cell membranes in contact with pond water. The greater the external TDS relative to internal TDS, the greater the pressure for ions to diffuse into cells and vice versa.
Resting Eggs of Fairy Shrimp and Their Hatching – top
How well did the “Rabbit Dry Lake” population do at adjusting its hatching rate to TDS? Brown and Carpelan (1971) did not graph or calculate statistics for the data but the raw numbers alone were impressive. More than 80% of eggs hatched at 1,000 micro-mhos or less and less than 1% hatched at 10,000 and 20,000 micromhos. There is more to the results than that though. The graph “Fairy Shrimp Hatching vs. Specific Conductance for Eggs from “Rabbit Dry Lake” ” shows that regression of the data points with the 3 lowest specific conductances in Table 4a of Brown and Carpelan (1971) has a very high coefficient of determination, or r-squared, of 0.9992. Thus, specific conductance explains essentially all of the variation in hatching rate. The correlation coefficient, which is the square root of the coefficient of determination, is significant at the 98% confidence level.
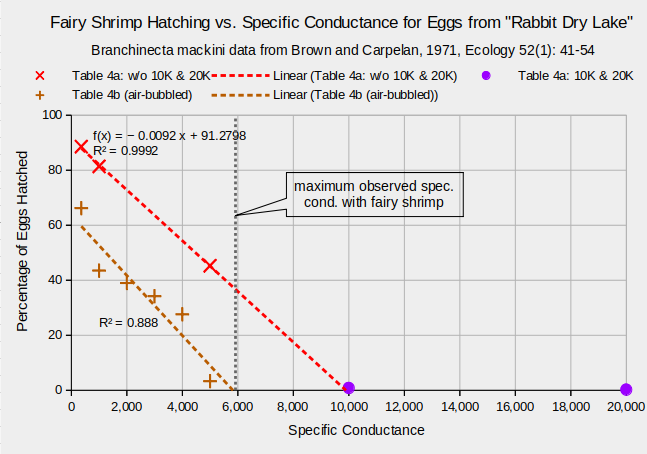
The hatching percentages at 10,000 and 20,000 micro-mhos are not included in the regression because these specific conductances are much higher than the highest observed by Brown and Carpelan (1971) in natural ponds, which was 5,850 micromhos (TDS 3,580 mg/L) in the 3 liters that remained of the summer 1966 pond. Moreover, very low hatching rates are prone to error due to the small number of hatches. 7 of 827 eggs hatched at 10,000 micro-mhos and 2 of 842 at 20,000 micro-mhos. In 6 of 7 trials at 20,000 micro-mhos, no eggs hatched. It is certainly tempting to include the 10,000 micro-mhos data point in the regression because that would increase the level of confidence to 99.9%. But including the 20,000 micro-mhos data point reduces the coefficient of determination to 0.7994 and the confidence level to 95%. The relation between hatching rate and specific conductance need not be linear at 10,000 micro-mhos and greater. It may be that the very low hatching rates at 10,000 and 20,000 micro-mhos represent the long tail of an asymptotic relation.
Brown and Carpelan (1971) were concerned that the eggs resting on the bottom of a petri dish during the experiments would experience microenvironments of low oxygen concentrations and that would distort the results. Consequently, they also reported, in Table 4b, the results of trials where air was continuously bubbled over the eggs. Air-bubbling reduced the hatching rates from 88% to 66% at 360 micro-mhos and from 45% to 3.3% at 1,000 micro-mhos. The slope of the linear regression for Table 4b data is similar to that for the Table 4a data (-0.0109 vs. -0.0092) but the intercept at 0 specific conductance is quite a bit lower. The level of confidence for the correlation coefficient of the Table 4b data is 99%. Whether the Table 4a or the Table 4b data are more realistic, both indicate that the “Rabbit Dry Lake” population is very good at using TDS to control hatching. It is intriguing that the Table 4b regression points to a 0% hatch at the maximum pond TDS observed by Brown and Carpelan (1971).
Resting Eggs of Fairy Shrimp and Their Hatching – top
Whatever their method, the “Rabbit Dry Lake” fairy shrimp eggs did even better on the playa. Brown and Carpelan (1971) reported their observations of 3 summer ponds with dramatically different durations and hatching rates.
- The August 1965 pond lasted 18 days and had a “large” but uncounted hatch. This pond duration was long enough for essentially all of the fairy shrimp to reach sexual maturity, which occurs when the fairy shrimp reach lengths of about 10 mm. In this pond, 17% of a collected sample was 19 mm long or longer on the 10th day after inundation.
- The August 1966 pond lasted 3 days. 74 nauplii were recovered from all the water that could be scooped up 12 hours before the last bit evaporated.
- The September 1967 pond lasted 5.3 days. Only 24 fairy shrimp were recovered from the water that was “siphoned” off the playa shortly before final desiccation.
For context, “Rabbit Dry Lake” has at times supported populations estimated at 400,000 fairy shrimp (Brown and Carpelan, 1971), although that may have been for a long-lived winter pond. The egg reservoir must be at least that big. 74 nauplii is a very small percentage of that.
For all 3 summer ponds, the initial specific conductances were about 1,000 micro-mhos. That specific conductance resulted in 80% of eggs hatching in the lab. In the real world, the “Rabbit Dry Lake” egg population didn’t fall for the 2 false promises. In the ponds that lasted for less than 6 days, only tiny percentages of eggs hatched. What was different between the laboratory and real world settings was the rate of change of TDS or its proxy, specific conductance. The rate of change was presumably zero in the lab. For the August 1966 pond, the specific conductance increased 5 times faster than in the August 1965 pond (Brown and Carpelan, 1971). Due to the linear relation, TDS would have increased at the same rate. The inert fairy shrimp eggs not only sense TDS, they have a sense of time and some way to remember earlier TDS.
A further demonstration of the “Rabbit Dry Lake” eggs ability to perceive and act in response to TDS comes from dilution experiments. Brown and Carpelan (1971) noticed several examples of hatching in response to an influx of water into an existing pond, including that from the melting of surface ice. In the lab, lowering TDS by dilution triggered hatching almost regardless of starting concentration and the greater the dilution, the greater the hatch. At the high end, dilution of TDS by 80% resulted in 80-90% of eggs hatching in waters with specific conductances of 3,000-9,000 micro-mhos. At the low end, 10% dilution resulted in about 50% hatching in water with specific conductance of 3,000 micro-mhos (1,910 mg/L TDS) and about 35% in 6,000 micro-mhos and 9,000 micro-mhos water (3,670 and 5,440 mg/L TDS) (Brown and Carpelan, 1971. Figure 7).
The “Rabbit Dry Lake” eggs are not alone in TDS astuteness. Fairy shrimp in rock pools in South Africa (n=15) did not hatch after 2 small early showers which resulted in pools with high initial TDS, as indicated by average electrical conductivities greater than 100 micro-Siemens per centimeter* (Vanschoenwinkel and others, 2010). Those populations did hatch in response to dilution caused by a subsequent heavy rain. Average pool conductivities decreased from 236 to 45 micro-Siemens per centimeter, which is a dilution of about 80%. Not all populations had perfect scores. Some eggs hatched in a few pools which started out with average conductivity of 40 micro-Siemens per centimeter but nonetheless dried up before any fairy shrimp matured. Numerically, Vanschoenwinkel and others (2010) observed a significant negative correlation between initial conductivity and pond duration (Spearman rank correlation -0.62, 99% confidence level).
* The units of conductivity are micro-Siemens per centimeter rather than micro-Siemens because values are not referenced to a standard as in specific conductance. Vanschoenwinkel and others (2010) did not provide the information needed to convert these values to TDS but my guess is that TDS is less than 500 mg/L even at 236 micro-Siemens per centimeter. That these fairy shrimp are not hatching in what would almost certainly be livable waters indicates they really are focused on pond duration and not physiological impacts of TDS.
Resting Eggs of Fairy Shrimp and Their Hatching – top
In the examples above, the hatching strategy for these fairy shrimp populations in low TDS water is to have more eggs hatch when the initial TDS concentration is low and the resulting pond is likely to last longer. Not all the eggs hatch at low TDS though due to the inherent uncertainty of ephemeral pond conditions. If the subsequent weather were hotter than what is normal in the collective memory of the population, the pond could dry up before any fairy shrimp reach maturity. A herd of thirsty horses could also dry up the pond unexpectedly (e.g., Wild Horse Rock Pool, Granite Mountains). Eggs are produced in excess of what is normally necessary for a successful annual hatch. Holding some eggs unhatched even under what appear to be good hatching conditions is cheap insurance in case things go bad. Conversely, it is advantageous to have some eggs hatch even under what appear to be poor hatching conditions in case things get better. Hatching rates consequently vary over wide ranges of TDS and are well tuned to local conditions in the “Rabbit Dry Lake” and South African rock pool populations.
Lower hatching rates at higher TDS is probably a different story for Artemia monica. “Mono Lake” doesn’t dry up and TDS probably doesn’t increase much with evaporation over the summer due to the large lake volume and relatively cool temperatures (possibly not much above 20 C, Jones & Stokes Associates, 1993b, p. J-3). The negative correlation of hatching rate and TDS for A. monica may be due to physiological stress.
Resting Eggs of Fairy Shrimp and Their Hatching – top
Oxygen Concentration Effects on Fairy Shrimp Hatching
Low oxygen concentrations inhibit hatching. When the oxygen concentration in the water holding Branchinecta mackini eggs from “Rabbit Dry Lake” was reduced from a normal level of 7 mg/L to 1 or 2 mg/L, no eggs hatched (Brown and Carpelan, 1971). The unhatched eggs were viable though as 60-70% hatched when subsequently exposed to oxygen-rich water. Oddly, eggs exposed to very low levels of oxygen for 120 hours and then normal levels hatched at a greater rate than eggs not exposed to low oxygen (Brown and Carpelan, 1971). Very low oxygen concentrations may thus precondition eggs for hatching.
Ephemeral ponds may experience large diurnal changes in oxygen concentrations with extremely low levels at night (Belk and Cole, 1975). This reflects the lack of photosynthesis by algae and the oxidation of organic matter by bacteria. Would fairy shrimp eggs be more likely to hatch in the morning as a consequence?
Initial flooding of a pond is typically accompanied by a low oxygen concentration as bacteria burst into action on the organic matter left over from the previous wet phase (Belk and Cole, 1975). Such low oxygen concentrations accompanying initial flooding could cause delays in hatching but would be difficult to identify in the field due to the similar effects of low temperatures.
“Mono Lake” in California is stratified with a lower water layer that has a very low concentration of oxygen. The resting eggs sink and those that land in the extensive low-oxygen sediment hatch at very low rates. In 1985, when the lake remained stratified through the winter, traps set at a depth of 7 m showed hatching rates of 720-25,340 nauplii per square meter per day and those set at 21 m depth showed hatching rates of 3-138 (Jones & Stokes Associates, 1993b, p. J-6). On the uncommon occasions when the lake turns over in winter (Jones & Stokes Associates, 1993b, p. J-5), the increase in oxygen concentration at the bottom of the lake would allow the hatching of eggs in the deeper water that hadn’t hatched in years.
Resting Eggs of Fairy Shrimp and Their Hatching – top
Carbon Dioxide Concentration Effects on Fairy Shrimp Hatching
An increase of carbon dioxide concentration coincided with hatching of Eubranchipus grubii (Rogers, 2013; Siphonophanes grubei in original) in a lab experiment as long as sufficient oxygen was present (Mossin, 1986, abstract only).
Resting Eggs of Fairy Shrimp and Their Hatching – top
Light Effects on Fairy Shrimp Hatching
Lighting affects hatching but is probably not a hatching trigger. Fairy shrimp can handle a wide range of lighting conditions. Laboratory experiments that tested fairy shrimp in a variety of other ways have kept the test animals under a range of lighting schemes: constant lighting (Atashbar and others, 2012), 12 hours light and 12 hours dark (Rogers, 2015), “natural” lighting (Beladjal and others, 2003), and 18 hours light with 6 hours dark for Antarctic fairy shrimp (Peck, 2004).
In experiments specific to lighting, fewer Artemia eggs hatched when kept in complete darkness for 5 days than when exposed to 1 hour of light at the beginning of the experiment (Sorgeloos (1973). For 3 different Artemia populations, the percentages of total eggs hatched for the light and dark treatments were 40% and 26%, 73% and 48%, and 95% and 62%, respectively. Whether the light source was a low intensity fluorescent tube or high intensity light bulb made no difference. Some of the unhatched eggs in the dark treatment did hatch when later exposed to light (Sorgeloos, 1973). The fact that in all 3 populations, the percentage that hatched in the dark is 65% of the percentage that hatched in the light is a puzzling consistency. Vanhaecke and others (1981) speculated that rather than light being a hatching trigger, darkness could be an inhibitor. This would be beneficial if there is an evolutionary advantage for buried eggs not to hatch when they get wet.
The eggs of Thamnocephalus platyurus hatched in greater percentages when exposed to longer periods of light (4, 8, 12, 16, and 24 hours) before being returned to the dark (Murugan and Dumont, 1995). Those exposed to continuous light during hydration hatched the most. Only 8% of eggs kept in total darkness hatched (Murugan and Dumont, 1995). In addition, a 12-hour light / 12-hour dark cycle resulted in a lower hatching rate than continuous illumination (Murugan and Dumont, 1995). Although these and Sorgeloos’s (1973) experiments are not entirely comparable, darkness seems to be more of an inhibition for T. platyurus eggs than for Artemia eggs.
Aside from light duration, light intensity didn’t seem to have much effect on hatching in one experiment but did in another. For Artemia populations at Buenos Aires and “Great Salt Lake”, the percentage of eggs hatched after 28 hours is between 95% and 100% regardless of light intensity varying from 20 lux to 2,000 lux at 5 treatment levels (Vanhaecke and others, 1981). The population at “Chaplin Lake” (Canada) was the exception to these results. After 34 hours, hatching percentages were only about 10% at 20 lux but up to 80% at 100 lux. Thamnocephalus platyurus eggs exposed to continuous 2,500 lux light during hydration hatched at a rate of 63% whereas those exposed to continuous 1,500 lux hatched at a 48% rate Murugan and Dumont (1995).
What seems to have been left out of the discussion of the effects of light on hatching are species that live in opaque waters. In the clay-rich water of “Rabbit Dry Lake” in the Mojave Desert, “[a]t no wave length does light penetrate below 20-25 mm” (Brown and Carpelan, 1971). Initial inundations of playa ponds are routinely deeper than 25 mm and, due to the winds that commonly accompany rain and snow storms, the water is usually opaque from the beginning. Presumably, Branchinecta mackini, Branchinecta gigas (both at “Rabbit Dry Lake”, Brown and Carpelan, 1971), and other species that live in opaque waters don’t need light to hatch. For them, light could indicate the water is too shallow and will dry up too quickly. Nonetheless, illumination apparently didn’t inhibit hatching in the experiments of Brown and Carpelan (1971). They didn’t specify the temperature or lighting regime of the hatching experiments so it is highly unlikely they kept the eggs in the dark.
Resting Eggs of Fairy Shrimp and Their Hatching – top
Other Controls on Fairy Shrimp Hatching
Elapsed time may be a hatching control. The purported requirement of Artemia monica to be exposed to cold water for 3 months was mentioned above in the temperature section. Elapsed time could also play a role in delaying hatching of A. monica at low temperatures. The average time to hatching was 42 days at 2.5 C and 3 days at 20 C (Jones & Stokes Associates, 1993b, p. J-4). As the experimental conditions were constant, only the passage of time determined hatching. In this case, slower metabolism at lower temperatures is a ready explanation. Is metabolism also a clock in other hatching processes, such as the change in hatching period from 1 day at high TDS to 38 days at low TDS (Brown and Carpelan, 1971) (mentioned in How Long Does Fairy Shrimp Hatching Last? above)?
Control by the passage of time could also explain how some eggs hatch only after they have been inundated several times. As many as 3 times for some Chirocephalus diaphanus eggs in Italy and 9 times for Streptocephalus vitreus eggs in Kenya (more below).
In some cases, the presence of adult fairy shrimp inhibits hatching of live eggs. In an insightful series of experiments, Beladjal and others (2007b) showed that non-dried eggs of Streptocephalus torvicornis didn’t hatch in water that had contained adult S. torvicornis. Non-dried eggs of Branchipus schaefferi didn’t hatch in water that had contained adult B. schaefferi. Eggs of both species hatched in water that had contained adult Chirocephalus diaphanus but at lower rates than in the controls. In an interesting twist, B. schaefferi hatched at low rates in water that had contained adult S. torvicornis but S. torvicornis did not hatch at all in water that had contained B. schaefferi. In explaining these results, Beladjal and others (2007b) noted that S. torvicornis and B. schaefferi commonly occur in the same ponds at the same time but do not occur with C. diaphanus. The muted inhibition of hatching in the presence C. diaphanus pheromones may be a generalized response to any adult fairy shrimp pheromone. Preventing eggs from hatching in ponds with adults could be beneficial to the population by preventing adults from ingesting newly hatched nauplii through normal filter-feeding (search for cannibalism on the Feeding by Fairy Shrimp page) and by avoiding depletion of food resources by a new generation (Beladjal and others, 2007b). That fairy shrimp eggs can detect pheromones left in the water by adult fairy shrimp and can distinguish pheromones of different species hints at a broad variety of cognitive skills for an embryo of a few thousand cells.
Hatching inhibition by parent pheromones may explain odd results in the experiments of Saengphan and others (2005) with Branchinella thailandensis eggs. Eggs retained in the parental water for 14 or 28 days hatched but none hatched when removed from the parental water on the day of release from the ovisac. Saengphan and others (2005) explained this as a requirement for B. thailandensis eggs to remain wet for at least 2 weeks in order to allow sufficient time for the embryo to develop to a hatchable stage within the egg. Alternatively, the work of Beladjal and others (2007b) described in the previous paragraph raises the possibility that the presence of pheromones inhibited hatching of eggs monitored immediately after release from the ovisac. If the pheromones broke down chemically in less than 2 weeks, then eggs left in the parent water for 14 and 28 days would not be inhibited.
Inhibition of hatching by adult fairy shrimp pheromones is not universal. Brown and Carpelan’s (1971) experiments with “ejected eggs” showed that Branchinecta mackini eggs from “Rabbit Dry Lake” hatched readily soon after release from the ovisac while remaining in the parental water.
Resting Eggs of Fairy Shrimp and Their Hatching – top
Hatching rate varies with clutch order for some fairy shrimp populations. Most female fairy shrimp produce more than 1 clutch of eggs. In some cases, eggs from early clutches do not hatch at the same rates as eggs from late clutches. With Chirocephalus diaphanus, early clutch eggs collected from young females hatched to a greater degree than late clutch eggs collected from old females (Zarattini, 2004). By experimental design, however, these effects were confounded with pond of origin and with cyclical dry-wet treatments. Nonetheless, as shown in the graph of “Effects of Clutch Order on Hatching of Fairy Shrimp Eggs Collected from 2 Ponds in Italy”, the percentages of hatching for each of the 3 dry-wet cycles and for the cumulative hatch were greater for the early clutches from both the semi-permanent “Tilia Lake” and the temporary “Illica Plain Pool”. Even so, because late clutches had more than 10 times more eggs than early clutches, the absolute number of eggs hatched was greatest for the late clutches.
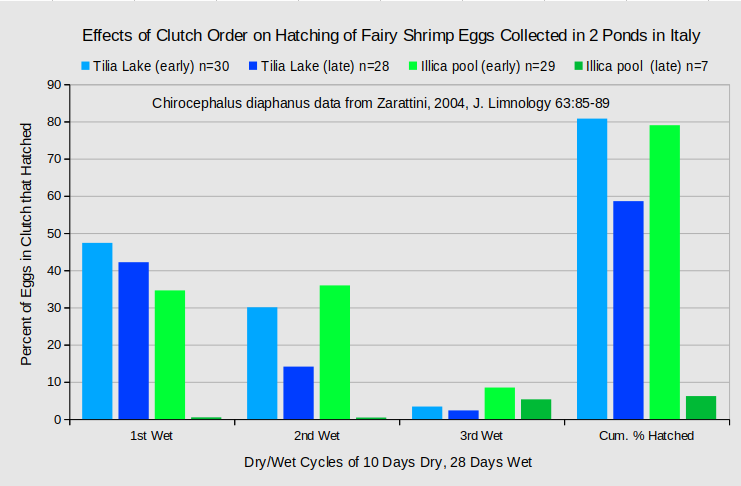
The relation of clutch order and hatching rate is not consistent across experiments. Early clutches showed lower hatching rates than late clutches in experiments with eggs of Branchinella thailandensis from a pond in Thailand (Saengphan and others, 2005). The hatching rates of the 1st, 6th, and 11th clutches of eggs from individual females of B. thailandensis were confounded with delays in removing the released eggs from the hatching water and with subsequent drying periods of different lengths but the 1st clutch eggs always hatched at lower rates than those of the 6th and 11th clutches (Saengphan and others, 2005). The graph of “Effects of Clutch Order on Hatching of Fairy Shrimp Eggs from Thailand Pond” further shows that 6th clutch eggs could hatch at higher or lower rates than 11th clutch eggs, depending on treatment.
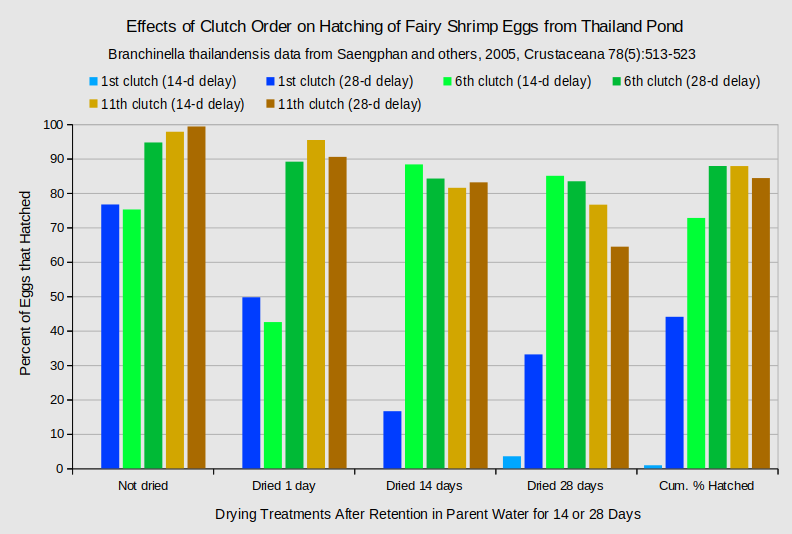
The variability of the parents’ physical environment may exert a control on egg hatching. This has been demonstrated using eggs of Chirocephalus ruffoi from Italy raised under indoor and outdoor conditions (Mura and Zarattini, 1999, abstract only). Temperature, light-dark cycle, and possibly other factors were held constant indoors but varied naturally outdoors. Eggs that were dried after collection and subsequently immersed in water hatched if they had been raised outdoors but not if they had been raised indoors (Mura and Zarattini, 1999, abstract only). Conversely, eggs that had been produced indoors hatched at higher rates than those produced outdoors when exposed to a dilution event or to a thermal shock. It was also noted that clutch sizes and life spans of females in the indoor and outdoor groups differed significantly (Mura and Zarattini, 1999, abstract only). One implication of this work is that results of laboratory experiments on fairy shrimp eggs cannot generally be used to predict hatching under natural conditions. They would need to be validated by observations of populations in the field.
Resting Eggs of Fairy Shrimp and Their Hatching – top
Randomization of Fairy Shrimp Hatching
Although it has been shown that various physical and chemical properties affect hatching, some hatching results seem to defy explanation. Hatching patterns are randomized rather than entirely predictable.
For example.
In the experiments of Brown and Carpelan (1971) (TDS above), 19% of Branchinecta mackini eggs didn’t hatch at TDS of about 730 mg/L when they would have been almost certain to survive in a natural pond.
In the experiments of Brown and Carpelan (1971), 2 of 842 Branchinecta mackini eggs hatched at TDS of about 12,000 mg/L even though TDS of about 6,000 mg/L on the 80th day of the 1966-1967 winter pond was deadly.
In the experiments of Murugan and Dumont (1995) (light above), 8% of Thamnocephalus platyurus eggs hatched in total darkness while all the others were apparently inhibited from hatching.
In the experiments of Zarattini (2004) (other controls above), some Chirocephalus diaphanus eggs hatched during the second or third immersions but not in the first under the same conditions.
In the experiments of Atashbar and others (2012), 27% of the eggs in 1 of 6 replicates did not hatch at the optimum temperature of 21 C but in another replicate only 3% did not hatch (mean of 85% and standard deviation of 12% for all replicates at that temperature and asssuming that at least 1 replicate hatched in accordance with maximum or minimum hatching rate indicated by the standard deviation).
In the experiments of Mura and Zarattini (1999, abstract only) (other controls), the hatching patterns of eggs produced by females raised under indoor or outdoor conditions varied widely and unpredictably. “Irrespective of the culturing conditions, the phenomenon of hatching was characterized by a very erratic pattern. An extremely high variation was observed within the clutches of a single female, as well as among different females. Such a variation was independent of female age, clutch order, and clutch size.”
Large variations in hatching rate over repeated cycles of drying and wetting provide additional examples of randomization. Among the 10 replicates of 100 Chirocephalus ruffoi eggs, each, for Pond 3, the range of hatching percentages in the 1st wet period was 1-36% (mean 9.1%), 2-46% (mean 14.9%) in the 2nd wet period, and 2-24% (mean 8.5%) in the 3rd wet period (Mura, 2001) (see graph “Randomization of Hatching for Fairy Shrimp Eggs from 2 Ponds in Italy”). The range was 8-65% over all 3 wet periods. Eggs in 10 replicates from an artificial Pool hatched at rates of 0-63% (mean 25.8%) during the 1st wet period, 1-29% (mean 9.8%) during the 2nd, and 1-28% (mean 7.7%) during the 3rd. The range was 7-78% over all 3 cycles. In other words, 93% of eggs never hatched in 1 replicate and all but 22% hatched in another (Mura, 2001).
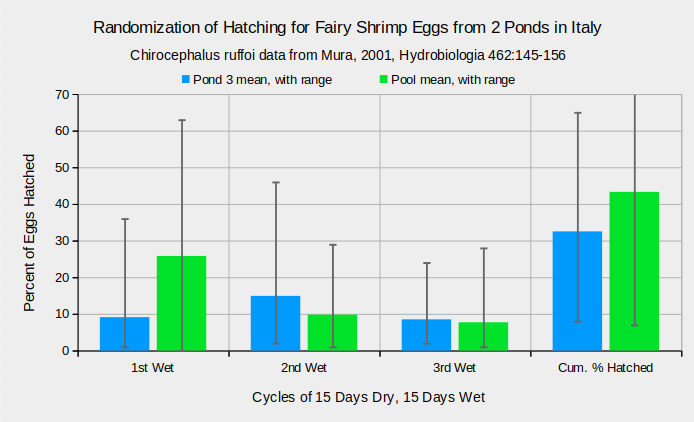
Chirocephalus diaphanus eggs from “Tilia Lake” and “Illica Pool” in Italy exhibited wide variations in hatching percentages between successive wet periods and between early and late clutches (Zarattini, 2004). 30 early clutches from “Lake Tilia” fairy shrimp had a mean clutch size of 42 eggs. The means (standard deviations) of eggs hatched for the 1st, 2nd, and 3rd wet periods were 20 (24), 12.5 (13.2), and 1.4 (5.0) eggs, respectively. Standard deviations even greater than the means indicate that neither statistic is particularly meaningful other than to indicate that the variations within wet periods were large. For “Illica Pool”, 29 clutches with a mean of 29.5 eggs hatched averages of 10.2 (11.3), 10.6 (14.4), and 2.5 (4.9) nauplii during the 1st, 2nd, and 3rd wet periods.
Resting Eggs of Fairy Shrimp and Their Hatching – top
Wide variations in hatching rates occurred over 9 cycles of 28 days dry – 28 days wet for eggs of Streptocephalus vitreus in Kenya. As illustrated by the graph “Randomization of Hatching of Fairy Shrimp Eggs Collected at 7 Distances from the Center of a Kenyan Rainpool”, variations for each distance sample over the 9 wet periods are large and trendless, even ignoring the near-zero hatches during the 1st and 6th periods (Hildrew, 1985). Some of the variations could be due to environmental segregation of the egg samples by distance from the pond center. Eggs deposited closer to the center of the pond could have developed in ponds of shorter duration and those nearer the periphery in ponds of larger sizes and longer durations. Or, eggs near the center could be from late clutches and those near the periphery from early clutches.
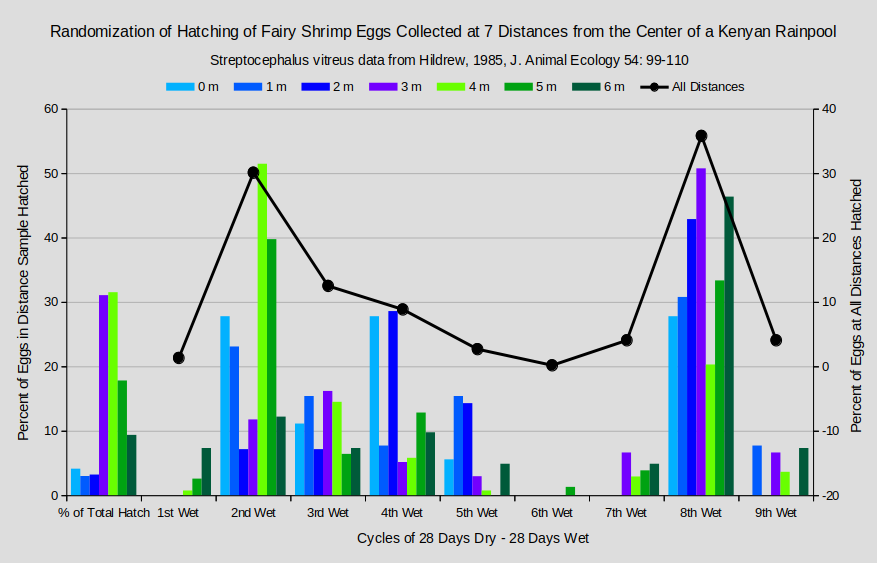
Range of eggs hatched in a sample: 13 to 138; total eggs hatched: 438.
The hatching patterns of eggs from the same clutch are just as erratic for the Kenyan fairy shrimp (graph “Randomization of Hatching of 11 Clutches from 11 Females of Fairy Shrimp from a Kenyan Rainpool”). Clutch H got off to a strong start with 15% hatching during the 1st wet period and 27% during the 2nd. But then no eggs hatched during the 3rd, 4th, 5th, or 6th wet periods. That was followed by 12% for the 7th wet period and 2% for the 8th. Clutch B followed a different pattern with hatching only during the 4th (3%), 5th (13%, 7th (17%), and 8th (7%) wet periods (Hildrew, 1985). The most consistent hatching pattern was for clutch F because only 1 egg (of 45) hatched for each of the 4th, 7th, and 8th wet periods. Not to mention the differences between clutches. No eggs of clutch A hatched but 56% of clutch H eggs did.
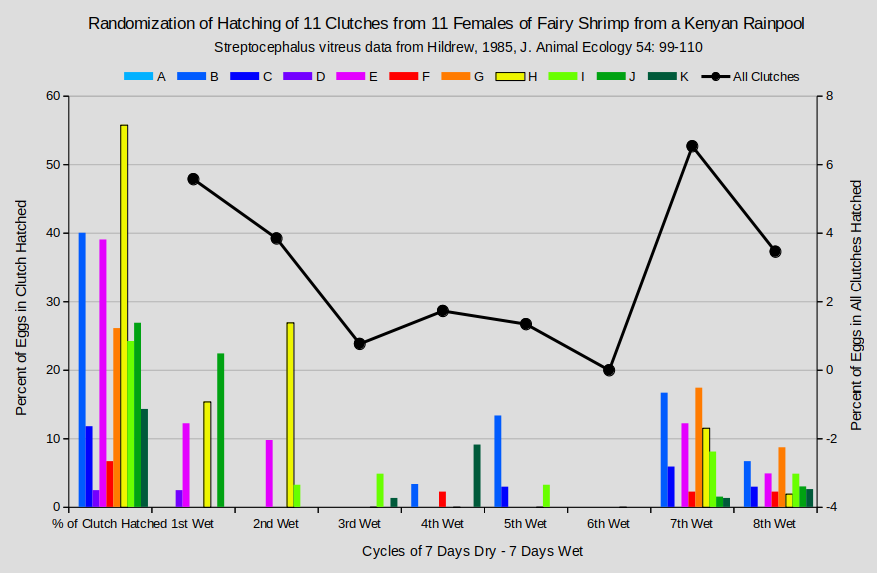
Range of clutch sizes: 25 to 77; total eggs: 520; total eggs hatched: 121.
Resting Eggs of Fairy Shrimp and Their Hatching – top
Traits other than hatching rate may also be subject to randomization. A good example is the huge range in clutch size and lifetime egg production of B. orientalis in the experiments of Atashbar and others (2012). For 6 replicates at each of 6 temperatures, average clutch sizes ranged from 14 to 109 and average lifetime egg productions from 24 to 1,094. Standard deviations were 14-27 and 23-329, respectively, resulting in coefficients of variation (standard deviation divided by average as percent) of 23-100%. Michaels and others (1988) citing Thompson (1984) indicated that coefficients of variation for traits in biological systems greater than 5% are considered large and found that the coefficients of variation of seed mass that they measured in 39 plant species were all large, 12-100%. Although the different experimental temperatures of Atashbar and others (2012) contribute to the variability of clutch size and lifetime egg production, even the lowest coefficients of variation, which occur for the 18 C trial, are 22% and 30%, respectively.
The examples of randomization above indicate that there are processes that affect hatching and other traits like clutch sizes that are non-deterministic. The results of these processes cannot be predicted from genetic, environmental, or other known variables. Biologists have lumped such processes under the term “developmental instability”. After investigating variations in leaf and flower traits in tobacco plants of different varieties, Sakai and Shimamoto (1965) developed a working definition: “The intragenotypic variability of quantitative characters which is considered to result from errors in developmental processes, though it might involve effects of indiscernible environmental influences, is called developmental instability”. Developmental instability is distinct from phenotypic plasticity, which is a common source of non-genetic variability. Phenotypic plasticity is “the ability of an individual organism to alter its physiology/morphology in response to changes in environmental conditions” (Schlichting, 1986, 1st page only). Even at constant experimental conditions, the variability of fairy shrimp hatching is quite large.
Rather than considering intragenotypic variability as errors, more recent research considers it noise, principally gene expression noise and transcription [of DNA] noise, which are often used interchangeably. DNA translation also affects gene expression but seems to be less written about. Genes typically produce proteins. In such a case, gene expression noise could be manifested as variations in the amount of protein produced per unit time or variations in the structures of the proteins produced. The unpredictability of the variations arises from the nature of the biochemical reactions that result in gene expression. Such reactions depend on reactants, transcription factors, ribosomes, RNA polymerases, and mRNA among other cellular components (Viney and Reece, 2013; Barroso and others, 2018; Urban and Johnston, 2018) which vary in abundances between genetically identical cells and between locations within cells in indeterminable ways. Consequently, gene expression can be hit or miss, depending on the immediate availability of required factors.
So what? In bacteria and yeast, transcription noise can determine whether cells survive antibiotic treatment, divide into new cells, or form spores in response to environmental stress (Pina, 2024). For example, when the abundance of the transcription factor ComK exceeds a certain threshold in Bacillus subtilis, the bacterium becomes “competent” and can take up DNA in order to survive a stressful condition (Urban and Johnston, 2018). Below that threshold, the bacterium remains “dormant” and less able to adapt.
Gene expression noise has physiological consequences in multicellular animals as well (Wolf and others, 2023). In mouse embryonic stem cells, increased gene expression noise results in an increased mutation rate and increased susceptibility to cancer (Pina, 2024). Higher levels of transcription noise in cancer cells is associated with greater severity of chronic lymphocytic leukaemia and with relapse in acute myeloid leukaemia (Pina, 2024).
Variable timing of seed germination is the archetype of high phenotypic variance (Simons, 2009). Environmental conditions of Simons’s (2009) germination experiments were essentially the same so he was not investigating phenotypic plasticity. Survival rates for the seeds of self-fertilizing Lobelia inflata planted by Simon (2009) ranged from 0.845 to 0.995 per day. (L. inflata is a widely distributed plant which prefers disturbed areas and is common in North America – illinoiswildflowers.info/savanna/plants/indian_tobacco.htm.) Those numbers aren’t that different but the consequences are “extreme”. At those rates, 50% mortality of seedlings occurred within 6 days or within 139 days (Simons, 2009). Simons (2009) did not specifically attribute the variability of survival rate to gene expression noise as his research had other objectives but the design of his experiments essentially eliminated genotypic and environmental variability as possible causes.
Resting Eggs of Fairy Shrimp and Their Hatching – top
It is not too much of a stretch, I hope, to suggest that the extreme variability of some fairy shrimp traits are due to developmental instability. The most suggestive case is the timing of hatching for eggs from the same clutch in the experiments of Hildrew (1985). Given controlled experimental conditions, phenotypic plasticity is not likely in play. The eggs of a clutch have the same parents but originated from different egg and sperm cells. This introduces the genotypic variability of siblings into the results. Refer again to Randomization of Hatching of 11 Clutches from 11 Females of Fairy Shrimp from a Kenyan Rainpool. Does the distribution of colors look like normal variation between siblings?
If the variability of hatching rates over different wet periods is due to genetics, one would expect the variability of eggs in 11 clutches from 11 different mothers to be less than the variability of eggs from thousands of potential mothers collected at 7 different distances from the center of the pond. Hildrew (1985) estimated the population size in a natural pool to be about 50,000 individuals on day 13 and about 10,000 individuals on day 25, when the fairy shrimp could all have been sexually mature. Eggs in the distance samples could have been deposited during several earlier pond fillings, adding even more mothers and fathers. In order to compare the variability of the clutch samples to the distance samples: 2 clutches which had no or only 1 egg hatch were excluded, the 9th wet period for the distance samples was excluded because the clutch samples had only 8 wet periods, the numbers of eggs hatching during each wet period were converted to percentages of all the eggs in that clutch or at that distance that hatched. This eliminated the effects of sample sizes ranging from 25 to 77 in the clutch samples and unknown numbers of eggs in the soil samples. Because no eggs hatched during so many wet periods, means, medians, standard deviations, and inter-quartile ranges are strongly influenced by the number of zero hatches and are uninformative. Consequently, the difference between the maximum hatching rate and the minimum hatching rate for each clutch or distance sample over 8 wet periods is used as a measure of variability here. Range differences are 31-83% hatching for the 9 clutch samples, with a median of 48%, and 28-51% hatching for the 7 distance samples, with a median of 43%. By this measure, the more genetically homogeneous clutch samples are more variable.
Greater, or at least not lesser, variability in the clutch samples suggests that the variability in hatching rate is not due to genetics and could be due to developmental instability. Variability due to phenotypic plasticity in response to different environmental conditions cannot be ruled out though. For example, experiments were conducted at room temperature, which no doubt varied somewhat. High hatching rates for most clutch samples during the 7th wet period and for distance samples during the 8th wet period do suggest changing experimental conditions. However, repeated measurements over 8 wet periods could average out differences in variability due to different environmental conditions. If developmental instability affected the experiments of Hildrew (1985), it could also have affected many of the other hatching experiments and many other fairy shrimp traits mentioned on this web site. As an intrinsic property of life, developmental instability affects all natural fairy shrimp populations and even you. Thanks to developmental instability all living individuals are unique.
The negative connotations of “instability” make developmental instability sound like a bad thing. However, to the extent that differences in gene expression noise result in differences in individual fitness, they are subject to natural selection (Viney and Reece, 2013; Barroso and others, 2018). Developmental instability has a purpose. For example, genes involved in different cell processes have different variances. Low variance genes are associated with functionally critical cell processes, such as RNA processing, translation, DNA methylation, DNA repair, and cell duplication. High variance genes are associated with maintenance of homeostasis, immune function, allergy, and responding to environmental factors (Wolf and others, 2023). The high variance allows for a greater range of outcomes and is a way to survive new allergens or diseases or changing environmental conditions. The consistent association of the variance of gene expression with particular cellular or individual-level processes or traits across species demonstrates that such variance is a fundamental pattern of life. Although it creates randomness, gene expression noise is not, itself, random.
Resting Eggs of Fairy Shrimp and Their Hatching – top
There is a cost to high variance. While it leads to better fitness in some individuals, it leads to poorer fitness and possibly early death in other individuals. This could explain why a few Branchinecta mackini eggs hatched in potentially deadly high-TDS water in Brown and Carpelan’s (1971) experiments, why some Thamnocephalus platyurus eggs hatched in total darkness (Murugan and Dumont, 1995), and why many eggs don’t hatch under optimum conditions in most fairy shrimp experiments.
How could developmental instability work in fairy shrimp? There is a possible analogy between the brown and black seeds of Suaeda salsa with different germination rates and fairy shrimp eggs with different susceptibilities to hydration. (S. salsa is a salt-tolerant, hermaphroditic annual that grows in estuary and lake shore wetlands.) Differential transcription in S. salsa results in either brown seeds, which germinate faster and tolerate higher salt concentrations, or black seeds, which remain dormant and tolerate lower salt concentrations (Gianella and others, 2021). The seed coats of brown seeds have lower phenolic contents. Consequently, they take up water more easily and germinate more quickly. The higher phenolic contents of black seed coats inhibits germination but promotes survival in water-logged intertidal soils (Gianella and others, 2021). Atriplex centralasiatica also has brown and black seed coats with different phenolic contents and different germination tendencies (Gianella and others, 2021). (A. centralasiatica is a salt-tolerant annual that prefers marshy soils.) Could gene expression noise in a fairy shrimp mother’s shell glands result in egg shells with different permeabilities? Eggs with less permeable shells might not fully hydrate the first (few) time(s) they are immersed and remain in the pond soil to hatch in some future year. If so, they would act as insurance against times when eggs hatch but the pond dries up before fairy shrimp mature.
Independent of any considerations of phenotypic plasticity or developmental instability, Grime and others (1981) had previously observed that “Although the role of impermeable seed coats under field conditions is still uncertain (Ballard 1973), there can be little doubt that this characteristic is often conducive to delayed germination and incorporation into persistent seed banks.”
Speculating further, there may be a high variance gene or genes in the mother that determine how much yolk an egg gets. That might determine how many hydration events an egg could survive without developing into an embryo and, hence, account for eggs that delay hatching through several inundation events. If eggs are sensitive to the amount of yolk they have left, they would be primed to hatch no matter what when the yolk reserve gets low. Eggs with lots of yolk could be picky.
Why would a mother vary the probability of hatching of its offspring by varying the yolk content of its eggs? As explained by Rees (1996) for plants, delayed germination benefits the parents in an evolutionary sense by reducing sibling competition. Also, in an environment that changes from year to year, a strategy of variable germination is optimal in the evolutionary sense because it allows some seeds to survive bad years. The same evolutionary reasoning applies to fairy shrimp eggs.
There is no evidence that I know of that fairy shrimp eggs of the same species have different amounts of yolk. There is abundant evidence they have different diameters. Different sizes could plausibly be related to different amounts of yolk. It just so happens that egg diameters are highly variable. A highly variable trait is not necessarily related to developmental instability but it could be. Using the graph of Hill and Shepard (1997), I have estimated the range and mean of egg diameters in millimeters for a few of the species with the largest ranges as follows.
- Branchinecta lindahli: 0.20-0.32, mean 0.24, range/mean=50%, n=89.
- Linderiella occidentalis: 0.20-0.34, mean 0.26, range/mean=54%, n=83.
- Streptocephalus texanus: 0.15-0.28, mean 0.24, range/mean=62%, n=21.
- Eubranchipus serratus: 0.26-0.49, mean 0.37, range/mean=62%, n=64.
- Branchinecta mackini: 0.15-0.32, mean 0.26, range/mean=65%, n=58.
- Branchinecta dissimilis: 0.15-0.34, mean 0.26, range/mean=73%, n=33.
Even dividing these range/means by 2 to make them more comparable to a coefficient of variation results in percentages greater than 25. Whether due to gene expression noise or something else, the large variability of egg diameters is telling us something. Maybe it is telling us whether an egg will hatch during its first inundation or during a later inundation.
The measurements of Hildrew (1985) do not indicate large variations in the sizes of the eggs in his experiments. He measured the mean diameter as 0.23 mm, with 95% confidence limits of 0.01 mm, and the mean dry weight as 2.8 micrograms, with 95% confidence limits of 0.6 micrograms. The eggs were apparently from an unspecified number of females collected for population and length measurements during periodic sampling of the long rains pool. The mean clutch size for these females was 42.1, with 95% confidence limits of 5.1. This confidence limit is only a third of the standard deviation calculated for the 11 clutches of eggs used in the second hatching experiment, with mean 47 and standard deviation of 16. Hildrew (1985) did not describe his method for calculating 95% confidence limits and it’s possible they actually underestimate the variability of egg sizes.
However it is accomplished, randomization of egg hatching traits serves a number of evolutionary purposes. Eggs that don’t hatch whenever inundated under favorable conditions aren’t a waste of parental resources. They build up an egg bank in the pond bottom soil that acts as insurance against population extirpation during years when none of the hatched fairy shrimp survive to reproduce. The accumulation of eggs of different generations over the years broadens the gene pool and that, in turn, may increase the adaptability of the pond population to changes in environmental conditions. Eggs that do hatch under unfavorable conditions are also not a waste of parental resources. They are lottery tickets. The probability is high that such hatchlings will die before reproducing but there is a chance they just might have the traits needed to survive under those conditions. The pay-off is sustaining the population through adverse conditions in the home pond. Or, if such eggs get dispersed, they could colonize a new pond with adverse conditions and extend the environmental range of the original population. Of one thing we can be certain, randomization of hatching is key to the survival of the anostracan order. If not, fairy shrimp wouldn’t do it. Whether humans can figure it out matters not a whit.
Resting Eggs of Fairy Shrimp and Their Hatching – top