Example Classification of Fairy Shrimp Habitats
Biological Constraints on Fairy Shrimp Habitats
pH of Fairy Shrimp Habitats
Temperatures of Fairy Shrimp Habitats
Total Dissolved Solids of Fairy Shrimp Habitats
Turbidity of Fairy Shrimp Habitats
Fairy Shrimp Osmoregulation and Thermoregulation
Example Classification of Fairy Shrimp Habitats
Biological, ecological, and morphological characterizations of fairy shrimp habitats have not been standardized and are often omitted from descriptions of fairy shrimp. On a global scale, they are also too diverse and wide-ranging to summarize here. However, results of an intensive survey of 5,565 ponds in California are too interesting to pass up. 26 wetland types were used to categorize fairy shrimp habitats. Fairy shrimp were found in 32% of the ponds, tadpole shrimp (Notostraca) in 17%, and clam shrimp (Conchostraca or order Spinicaudata in this case) in 2% (Helm, 1996, Figure 4, where categories are apparently mutually exclusive).
As an example of the variety of fairy shrimp habitats that can be distinguished in a specific region, Helm’s (1996) list of wetland types follows.
- Alkaline Pool – on alkaline soil, may have salt-tolerant plants.
- Artificial Seasonal Wetland – temporary, on grassland or fringing perennial wetlands or ponds, slow draining bottom, created inadvertently with earth-moving equipment.
- Artificial Vernal Pool – seasonal, relatively impermeable bottom, created inadvertently with earth-moving equipment.
- Clay Flat – flat-floored depression on clay-rich soil.
- Created Pool in Ephemeral Drainage – shallow check dam on ephemeral drainage constructed to replace natural pool.
- Created Vernal Pool – seasonal, excavated shallow depression to replace natural pool in vernal pool terrain, inoculated with topsoil from natural pool.
- Ditch – constructed drain.
- Dozer Scrape Vernal Pool – created inadvertently due to firebreak construction in vernal pool terrain.
- Ephemeral Drainage – shallow, sparsely or unvegetated water course with well defined banks that flows during and after rain.
- Fresh Water Marsh – semi-permanent or permanent water on saturated soil, dominated by emergent sedges and other vegetation.
- Percolation Test Pit Pool – steep-sided, rectangular pits dug with backhoe to test water percolation capacity of soil.
- Pond – semi-permanent or permanent, area less than 3.24 hectares (8 acres), depth greater than 2 m (6.6′).
- Railroad Right-of-Way Pool – shallow, elongated depressions adjacent to railroad tracks, common vehicular disturbance.
- Railroad Right-of-Way Vernal Pool – seasonal, vegetated, unlike Railroad Right-of-Way Pool.
- Road Rut Pool – constantly disturbed vehicle ruts in roads through vernal pool terrain.
- Road Rut Vernal Pool – seasonal, vegetated, unlike Road Rut Pool.
- Road Side Ditch – drainage ditches constructed parallel to roads.
- Road Side Vernal Pool – seasonal, ponding duration longer and depth greater than for Road Side Ditch.
- Rock Outcrop Pool – on exposed bedrock, soil 0-3 cm deep, may support very sparse vegetation.
- Seasonal Wetland – temporary, on grassland or fringing perennial wetlands or ponds, slow draining bottom, vegetation differs from that of Vernal Pool.
- Stock Pond – semi-permanent or permanent, dam in ephemeral or intermittent drainage, area less than 3.24 hectares (8 acres), depth usually greater than 60 cm (2′).
- Stream Oxbow – abandoned channel in meandering stream, fed by direct precipitation, surface flow, and groundwater.
- Vernal Lake – seasonal, same as Vernal Pool except area greater than 3.24 hectares (8 acres).
- Vernal Pool – seasonal, depression on poorly draining soil, distinctive vegetation adapted to dry – wet cycles, area less than 3.24 hectares (8 acres).
- Vernal Swale – seasonal, broad, shallow, poorly defined drainages on gently sloping topography, may connect Vernal Pools, may drain into ephemeral drainages.
Approximately two-thirds of the wetlands sampled were Vernal Pools (Helm, 1996). Vernal Pools was the most common wetland type for Branchinecta mesovallensis (93%) (called Midvalley Fairy Shrimp, “Branchinecta sp.” by Helm, 1996, and not formally named until later), Linderiella occidentalis (81%), Branchinecta lynchi (79%), and Branchinecta conservatio (68%). These results are not representative of California as Helm’s (1996) work was focused on the San Joaquin and Sacramento valleys and excluded southern California, northwestern California, and the Sierra Nevada.
Habitats of Fairy Shrimp – top
Different species of fairy shrimp were found in different wetland types but with considerable overlap between some species. Of the fairy shrimp species of interest to Helm (1996):
- Branchinecta longiantenna occurred in Alkaline Pools and Rock Outcrop Pools,
- Branchinecta conservatio occurred in Alkaline Pools, Vernal Lakes, and Vernal Pools.
- Branchinecta mesovallensis occurred in Vernal Pools and Vernal Swales,
- Branchinecta lynchi and Linderiella occidentalis occurred in at least 20 wetland types each, including Alkaline Pools, Seasonal Wetlands, Vernal Pools and Swales, Stream Oxbows, Ephemeral Drainages, Stock Ponds, Road Side Ditches and Vernal Pools, Railroad Right-of-Way Pools and Vernal Pools, and Road Rut Pools,
- Branchinecta lynchi also occurred in Rock Outcrop Pools and Dozer Scrape Vernal Pools, and
- Linderiella occidentalis also occurred in Fresh Water Marshes.
The presence of B. longiantenna in both Alkaline Pools and Rock Outcrop Pools is particularly notable. Waters in Alkaline Pools have higher pH and higher TDS than waters in Rock Outcrop Pools. “Alkaline” is just another way of saying high pH. “Salt-tolerant plants” and “sometimes salt encrusted” (Helm, 1996) are clear evidence of high TDS. B. longiantenna is a good candidate for a species with high phenotypic plasticity. By developing into different phenotypes based on different local environmental conditions, the species can adapt to different habitats. Alternatively, Helm (1996) thought there might be 2 subspecies adapted to the different conditions.
None of the 8 fairy shrimp species investigated by Helm (1996) were found in semi-permanent or permanent Ponds, although a clam shrimp species (class Branchiopoda, Conchostraca or order Spinicaudata in this case) was. Helm (1996) did not even consider a wetland type for lakes larger than 3.24 hectares (8 acres). Could that be because of fish?
The graph “Mean Depths and Maximum Areas of Ponds Inhabited by 5 Species of California Fairy Shrimp, With Ranges” illustrates the wide ranges of water depths and pond sizes that the California fairy shrimp live in. “Mean average potential depth” is the mean for all ponds in the category of the average of several measurements of depth below the “high water line” collected along a line across the pond (Helm, 1996). The “high water line” was estimated from the spill point of the pond. “Mean maximum potential pond area” is the mean for all ponds in the category of the surface area measured when ponds were at their greatest observed extents. For ease of visualizing the areas, a square with sides of 320 m (1,050′) has an area of 10 ha, a square with sides of 100 m (330′) is 1 ha, a square with sides of 32 m (105′) is 0.1 ha, a square with sides of 10 m (33′) is 0.01 ha, and a square with sides of 3.2 m (10.5′) is 0.001 ha.
The main points are that fairy shrimp live in ponds of many sizes and that there is extensive overlap in the sizes of ponds inhabited by different species of fairy shrimp. Although it may appear that B. conservatio generally lives in bigger ponds than B. mesovallensis, each species was found in only a small number of ponds. Those ponds may be flukes of rare colonization events and not representative of the species’ habitat possibilities.
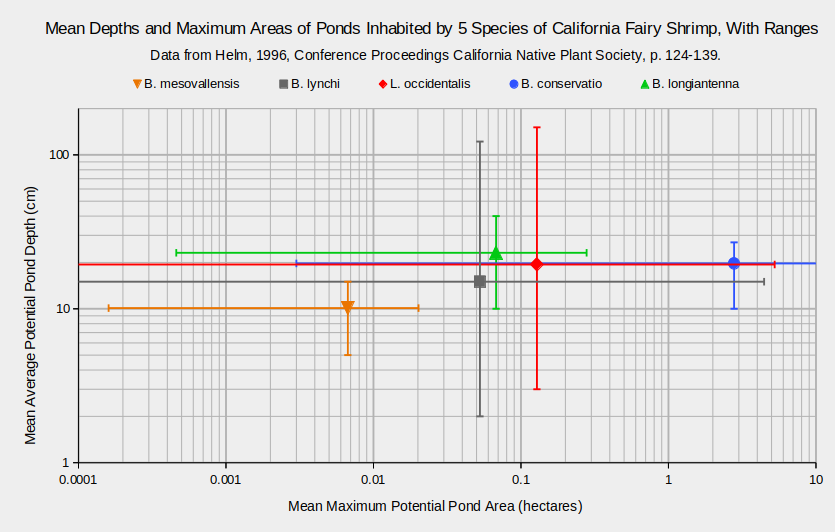
B. – Branchinecta, L. – Linderiella
Error bars represent ranges rather than standard deviations.
Numbers of natural ponds for depth/area measurements: 24/14 for B. mesovallensis, 605/697 for B. lynchi, 487/693 for L. occidentalis, 19/19 for B. conservatio, and 7/6 for B. longiantenna.
That fairy shrimp which mature faster generally inhabit smaller ponds than those which mature slower is an appealing idea. Obviously, a fairy shrimp species that takes a long time to mature won’t survive in ponds that dry up quickly. Using pond depth as a proxy for pond duration, the graph “Mean Days to Maturity vs. Mean Average Potential Pond Depth for 5 Species of California Fairy Shrimp” shows that the idea is too simplistic. L. occidentalis takes 33 days on average to reach maturity but it still survived and reproduced in 1 pond that has an average potential pond depth of only 3 cm. At the other end of the 5 species maturity spectrum, the fastest maturing species is not in any way excluded from deep ponds, as shown by the wide range of sizes for B. lynchi ponds. Using pond depth as a criterion for finding fairy shrimp in general or one species in particular is not a good idea. WIDLA.
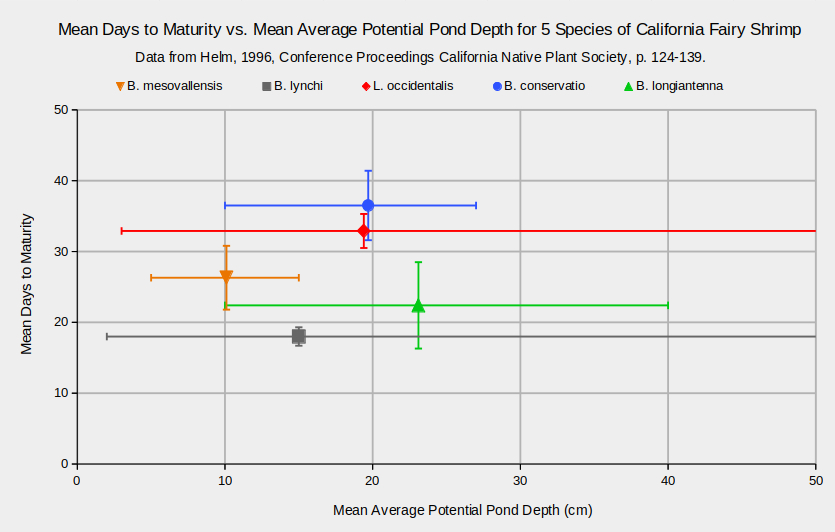
B. – Branchinecta, L. – Linderiella
Error bars represent ranges for average potential depths and standard deviations for days to maturity.
Days to maturity were determined from populations raised in plastic pools: 8 pools for B. mesovallensis, 24 for B. lynchi, 25 for L. occidentalis, 10 for B. conservatio, and 3 for B. longiantenna.
Plastic pools 140 cm in diameter were “seeded” with soil from ponds known to have fairy shrimp and allowed to develop in response to natural precipitation and temperatures. Any fairy shrimp that hatched were not fed. All pools had abundant tadpoles and many had predators, such as dytiscids, notonectids, and odonatans.
Much more information on California fairy shrimp habitats can be found in the 1999 book “Fairy Shrimps of California’s Puddles, Pools, and Playas” (Mad River Press, Eureka, CA) by C.H. Eriksen and D. Belk but I’ve only read reviews and excerpts. The book has information on water chemistry and probably several other relevant environmental conditions.
Habitats of Fairy Shrimp – top
There are many other ways to categorize ponds. For the ponds in northern Finland, principal component analysis of the variables elevation, surface area, maximum depth, temperature, pH, dissolved organic carbon, bottom type, and presence or absence of vegetation separated the ponds into 4 types, with minimal overlap (Rautio, 2001). The zooplankton communities differed between the different pond types.
- “Barren” ponds are characterized by the highest elevations (greater than 700 m, (2,300′), lowest TDS (mean electrical conductivity 5 microSiemens per centimeter), lowest dissolved organic carbon (mean 1.2 micrograms per liter), rocky bottoms, and little vegetation. The copepod Mixodiaptomus laciniatus and the cladoceran Acroperus elongatus were abundant in these ponds. The latter occurred in other pond types but the former did not. The fairy shrimp Polyartemia forcipata occurred in 4 of the 5 ponds (Rautio, 2001).
- “Tundra” ponds are above tree line and have abundant shoreline vegetation. They lie at 600-700 m (1,970-2,300′), between the barren ponds and the mountain birch woodland ponds. These ponds were dominated by the cladocerans Bosmina obtusirostris and Polyphemus pediculus to the exclusion of pretty much all other crustaceans. P. forcipata occurred in 4 of the 5 ponds (Rautio, 2001).
- “Mountain birch woodland humic” ponds are boggy and surrounded by Sphagnum mosses. The have the lowest pH (mean 5.9) and the highest dissolved organic carbon (mean 11 micrograms per liter) and chlorophyll-a. The cladoceran Daphnia longispina was abundant and occurred only in this pond type. 4 other cladoceran species and the copepod Microcyclops gracilis were also common. Polyartemia forcipata occurred in 2 of the 2 ponds (Rautio, 2001).
- “Mountain birch woodland clear” ponds have dense shoreline vegetation. Some have bottoms of thick algal mats. They have the highest TDS (mean electrical conductivity 40 microSiemens per centimeter) and second highest dissolved organic carbon and chlorophyll-a. Like the tundra ponds, these ponds were dominated by B. obtusirostris and P. pediculus with no other crustacean accounting for more than 3% of the community. P. forcipata was absent from all 5 ponds (Rautio, 2001).
The absence of P. forcipata from the mountain birch woodland clear ponds cannot be explained by differences in the observed physical, chemical, or biological conditions as they overlap those of some of the other ponds. Predation can’t account for the absence either. All the ponds were believed to lack fish. Insect predators of the orders Coleoptera (includes dysticids) and Heteroptera (includes backswimmers) were observed in all the ponds (Rautio, 2001).
Various physical and chemical conditions are commonly reported for fairy shrimp habitats. These include pH, temperature, and TDS. Although not commonly measured, turbidity is a visually obvious physical parameter that affects species distributions. Osmoregulation and thermoregulation are the biological processes fairy shrimp use to deal with TDS and temperature levels and their variations. But first a brief look at biological constraints on fairy shrimp habitats.
Habitats of Fairy Shrimp – top
Biological Constraints on Fairy Shrimp Habitats
Persistent fairy shrimp populations do not occur with year-round fish populations.
Over-wintering salamander populations can prevent fairy shrimp colonization (Bohonak and Whiteman, 1999). The same may be true for frogs.
The possible roles of other predators, such as amphipods and backswimmers, in limiting fairy shrimp populations are considered in their respective sections under Predators of Fairy Shrimp.
Fairy shrimp inhabit alpine lakes with very low phytoplankton concentrations. If there is a lower limit to the phytoplankton abundance needed for fairy shrimp to survive, I haven’t read of it.
The absence of an effective animal dispersal agent could be considered a biological constraint but is dealt with under Dispersal of and Colonization by Fairy Shrimp.
Habitats of Fairy Shrimp – top
pH of Fairy Shrimp Habitats
Acidity, as measured by pH, has not been considered an important control on fairy shrimp occurrence (e.g., Horne, 1967; Gonzalez and others, 1996) because most species can handle the pH range for most natural waters. At the extremes, however, few species are found in waters with pH greater than 10.0 or less than 5.0.
For context, the U.S. Environmental Protection Agency has set a drinking water secondary standard of 6.5-8.5 for pH due to the possibility of bitter metallic taste and corrosion at less than 6.5 and of soda taste and mineral deposits at greater than 8.5. Orange juices have pH’s of 3.3-4.2 (wikipedia).
The graphs of “Low, Median, and High pH of Waters With Fairy Shrimp that Occur in California” and “Low and High or Single Value of pH of Waters With Fairy Shrimp Outside California” summarize the pH ranges for ponds by species of fairy shrimp inhabitants.
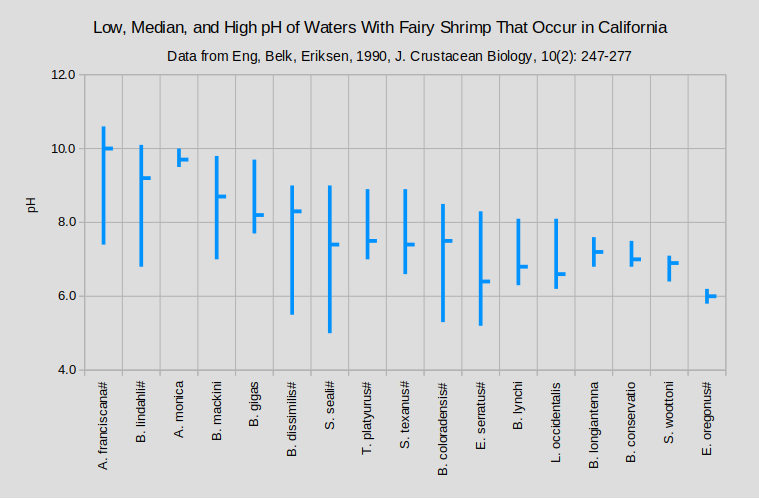
#Range includes ponds outside California; median is for ponds outside California.
A. – genus Artemia; B. – genus Branchinecta; E. – genus Eubranchipus; L. – genus Linderiella; S. – genus Streptocephalus; T. – genus Thamnocephalus
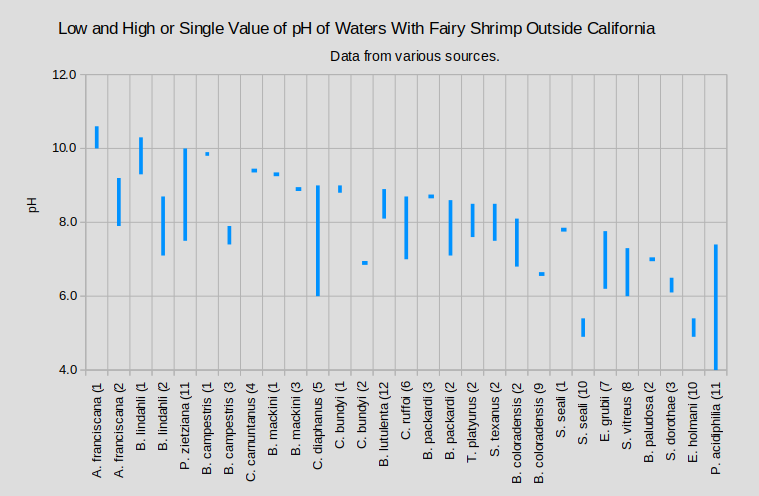
A. – genus Artemia; B. – genus Branchinecta; C. – genus Chirocephalus; E. – genus Eubranchipus; P. – genus Parartemia; S. – genus Streptocephalus; T. – genus Thamnocephalus
(1 McCarraher, 1970; (2 Horne, 1967; (3 Maynard and Romney, 1975; (4 Horvath and Vad, 2015; (5 Mura and others, 2003; (6 Mura, 2001; (7 Goldyn and Bernard, 2008; (8 Hildrew, 1985; (9 Moore, 1950; (10 Moore, 1963; (11 Timms, 2011; (12 Rogers and Hill, 2013a
Habitats of Fairy Shrimp – top
Temperatures of Fairy Shrimp Habitats
Fairy shrimp inhabit the tropics and the poles but different species are more commonly found in cooler or warmer environments (e.g., Belk, 1977). Describing the temperature preferences of species is complicated by the wide ranges of temperatures most populations have to deal with in their normal life cycles. Ponds heat up during the summer. Ephemeral ponds heat up more as they get shallower. In some environments, daily water temperature changes can exceed 10 C (e.g., Peck, 2004). In most cases, reported temperatures have not been monitored continuously and consequently underestimate the true ranges of tolerable pond temperatures.
The graphs “Low and High or Single Value of Temperatures of Waters With Fairy Shrimp That Occur in California” and “Low and High or Single Value of Temperatures of Waters With Fairy Shrimp Outside California” show that most species tolerate wide ranges in temperature.
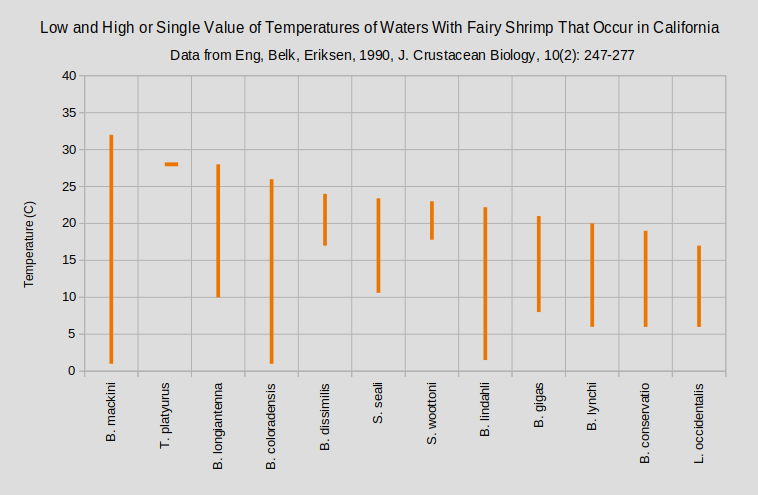
A. – genus Artemia; B. – genus Branchinecta; L. – genus Linderiella; S. – genus Streptocephalus; T. – genus Thamnocephalus
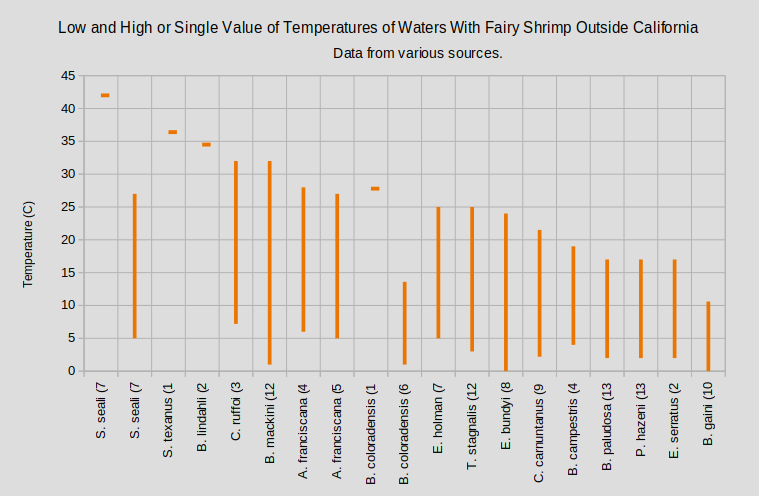
A. – genus Artemia; B. – genus Branchinecta; C. – genus Chirocephalus; E. – genus Eubranchipus; P. – genus Polyartemiella; S. – genus Streptocephalus; T. – genus Tanymastix
(1 Moore, 1950; (2 Belk, 1977; (3 Mura, 2001; (4 Broch, 1969; (5 Wurtsbaugh and Gillie, 2001; (6 Shantz, 1905; (7 Moore, 1963; (8 Daborn, 1976; (9 Horvath and Vad, 2015; 10) Jurasz and others, 1983; (11 Brown and Carpelan, 1971; (12 Mura and Zarattini, 2000; (13 Miller and others, 1980, p. 56
At what water temperatures did they die?
“LD50” = lethal dose for 50% of the experimental population
- 26-28 C (2-4 hours) – Eubranchipus holmani (Moore, 1963),
- 30 C (30 minutes) – Eubranchipus holmani (Moore, 1963),
- 32 C (1 hour, after acclimation at 13.5 C) – Eubranchipus bundyi females (males died at 29) (“LD50” of Belk, 1977),
- 32 C (1 hour) – Eubranchipus serratus females (males died at 30) (“LD50” of Belk, 1977),
- 32 C (1 hour, after acclimation at 13.5 C) – Branchinecta paludosa females (“LD50” of Belk, 1977),
- 36 C (1 hour) – Branchinecta lindahli females (“LD50” of Belk, 1977),
- 38 C (1 hour) – Branchinecta packardi females (males died at 36 C) (“LD50” of Belk, 1977),
- 38 C (1 hour) – Streptocephalus seali (“LD50” of Belk, 1977),
- 40 C (1 hour) – Streptocephalus dorothae (“LD50” of Belk, 1977),
- 41 C (1 hour) – Streptocephalus mackini (“LD50” of Belk, 1977),
- 41 C (1 hour) – Thamnocephalus platyurus (“LD50” of Belk, 1977),
- 43-44 C (30-minute exposure, 18 hours until death) – Streptocephalus seali (Moore, 1963),
It may be a general rule in fairy shrimp that at high temperatures, they grow quickly and die early and at low temperatures, they grow slowly and live longer, albeit with large variations between species and populations.
Habitats of Fairy Shrimp – top
The trade-off between growth rate and life span is illustrated by the graph of “Fairy Shrimp Growth Rates vs. Temperatures for Eggs Collected in Iran”. Growth rates increased rather steadily from 12 C to 27 C (Atashbar and others, 2012). By the 21st day of the experiment, more than 80% of the fairy shrimp at 12 C, 15 C, and 18 C were still alive but only 26% of those at 24 C and none of those at 27 C (Atashbar and others, 2012).
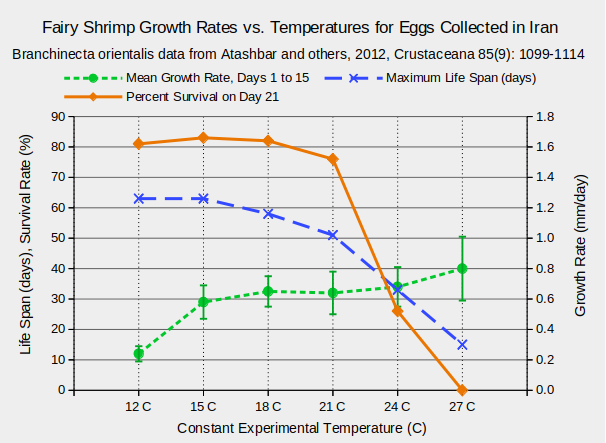
The experiment ended on day 63; observations were generally every 6th day.
Similarly, experiments with Streptocephalus seali found a maximum growth rate of 1 mm/day (pre-adult) at 34 C but a maximum life span at 19 C (Anderson and Hsu, 1990, abstract only). Higher growth rates coincided with faster maturation. Females became mature within a mean of 12 days at 32 C and 17 days at 27 C (Anderson and Hsu, 1990, abstract only).
Antarctica is certainly one of the cooler fairy shrimp habitats. There, Branchinecta gaini nauplii, which may have been a few days old, were observed on December 2, 1977 (Jurasz and others, 1983). By January 2, 1978, females with eggs were up to 12 mm long (growth rate 0.39 mm/day) and the longest male was 16 mm (growth rate 0.52 mm/day). None of the fairy shrimp in this population grew to be more than 20 mm long. These slow growers lived for about 6 months (assuming the generation observed from November 11, 1977 to March 2, 1978 lived as long as the previous generation, which was last seen on May 19, 1977; Jurasz and others, 1983).
Laboratory experiments with Branchinecta gaini adults collected live from ponds on Anchorage Island, Antarctica, indicated death due to high water temperatures may be caused by oxygen deficiency. Temperatures in the ponds inhabited by the fairy shrimp did not exceed 20 C over 1 year of monitoring (Peck, 2004). In the experiments, no fairy shrimp died over a period of 1 week at 10 C. At 25 C, the dying began within 10 hours. 50% of the test animals had died after 47 hours and 100% had died after 80 hours. At 30 C, none of the fairy shrimp survived for more than 5 hours (Peck, 2004).
Oxygen consumption by the fairy shrimp increased with temperature and so did the variance of oxygen consumption (Peck, 2004). Mean oxygen consumptions (n=22 to 32) at 1 C, 10 C, and 20 C were 0.093, 0.176, and 0.37 micromoles per animal-hour. As an indication of the variance, the maximum consumption at 20 C was 0.66 micromoles per animal-hour, nearly a factor of 2 higher than the mean. At 25 C, mean oxygen consumption declined. All the males and some of the females died.
Peck (2004) observed that the beat frequencies of the fairy shrimps’ legs increased with temperature. Beat frequencies were generally higher for females than for males, which were less able to survive higher temperatures. In females, the mean (n=20) beat rate increased from 99 beats per minute at 1 C to 143 beats per minute at 10 C (Peck, 2004). Peck’s (2004) implication is that fairy shrimp increased their leg motion in order to obtain more oxygen. This may be so obvious that it does not need to be explained, except to me. However, leg motion is also feeding. Do fairy shrimp increase the frequency of leg beating in order to increase oxygen consumption in response to a temperature increase or does oxygen consumption increase because the legs are beating faster in order to ingest more food to support a higher metabolic rate at higher temperature?
Peck (2004) did not feed the fairy shrimp in his experiments. Instead, he placed about 1 cm of sediment from the bottom of the home pond into each beaker of experimental animals and refreshed the water periodically with water from the home pond. If the experimental water did not have floating plankton, then the fairy shrimp would have had to scrape the sediment at the bottom of the beaker in order to obtain food. In that case, increasing their leg beating frequencies while filter feeding would not result in greater food ingestion.
Temperature also affects egg production. In experiments with Branchinecta orientalis, clutch sizes and total egg production per female increased from 12 C and 15 C to an optimum at 18 C and then decreased. See the graph of “Fairy Shrimp Egg Production vs. Temperatures for Eggs Collected in Iran”.
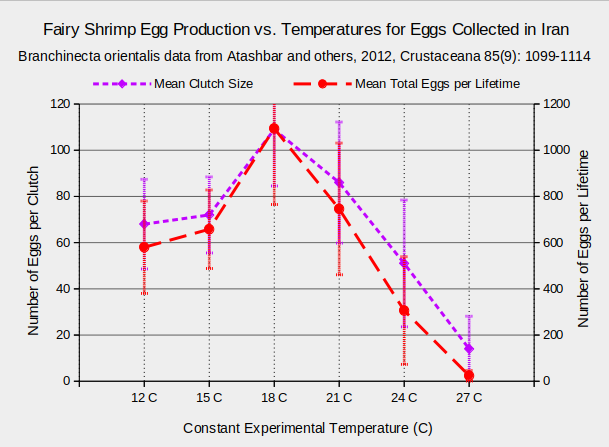
The experiment ended on day 63; observations were generally every 6th day.
Habitats of Fairy Shrimp – top
Total Dissolved Solids of Fairy Shrimp Habitats
Total dissolved solids (TDS, see the Acronyms page for explanation) is one control on which ponds particular species inhabit. Because some populations of the genera Artemia and Parartemia inhabit waters with TDS more than triple the TDS of seawater, the TDS range for the order Anostraca is very wide. For context, the secondary drinking water standard for TDS in the United States is 500 mg/L due to possible unpleasant aesthetic effects above that concentration. Seawater has TDS of about 35,000 mg/L.
In addition to populating habitats with a huge range of TDS, individual fairy shrimp can survive large changes in TDS, as they must do if their pond is drying up. Branchinecta lindahli, Branchinecta packardi, Streptocephalus texanus, and Thamnocephalus platyurus survived increases of TDS from 1,100 mg/L to 4,600 mg/L over a 7-day period in the laboratory (Horne, 1967). B. lindahli and S. texanus also survived increases of TDS from 9,500 mg/L to 16,500 mg/L over a 3-day period in the pond (Horne, 1967). In a winter pond in the Mojave Desert, Branchinecta mackini survived an increase in TDS from less than 400 mg/L to about 1,700 mg/L over a period of about 56 days (Brown and Carpelan, 1971, Fig. 4).
The graphs of “Low, Mean, and High TDS of Waters With Fairy Shrimp That Occur in California” and “Low and High or Single Value of TDS of Waters With Fairy Shrimp Outside California” show that the ranges of tolerable TDS by species are quite large and overlapping. As in the cases of pH and temperature, reported TDS concentrations underestimate the full range of TDS of the waters in which fairy shrimp live. Samples are rarely collected as soon as the fairy shrimp hatch or on the day the last one dies. Moreover, electrical conductivity is used much more commonly than TDS as a measure of water quality or salinity because it is easier to measure.
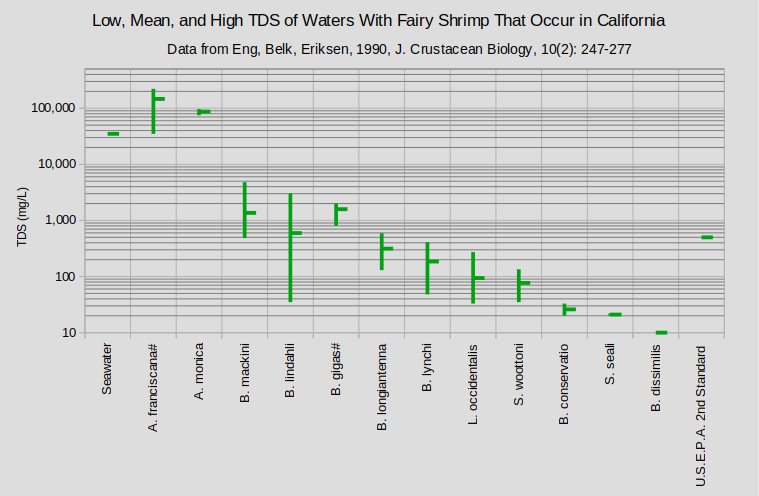
#Range includes ponds outside California; median is for ponds outside California.
A. – genus Artemia; B. – genus Branchinecta; L. – genus Linderiella; S. – genus Streptocephalus
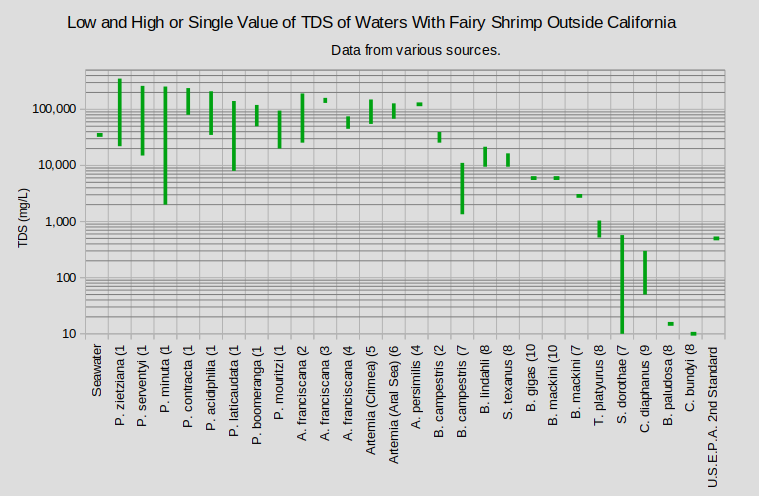
A. – genus Artemia; B. – genus Branchinecta; C. – genus Chirocephalus; P. – genus Parartemia; S. – genus Streptocephalus; T. – genus Thamnocephalus
(1 Timms, 2011; (2 Broch, 1969; (3 Wurtsbaugh and Gillie, 2001; (4 de los Rios and Salgado, 2012; (5 Anufriieva and Shadrin, 2019; (6 Marden and others, 2012; (7 Maynard and Romney, 1975; (8 Horne, 1967; (9 Mura and others, 2003; (10 Brown and Carpelan, 1971
Considerable research on the effects of TDS on fairy shrimp has been conducted using Artemia species because of their economic importance as fish food. Various life history characteristics are affected by TDS. For Artemia urmiana and A. parthenogenetica in Lake Urmia, Iran, body lengths and survival rates 20 days after hatching generally decreased at TDS concentrations greater than 125,000 mg/L (Agh and others, 2008) (see graphs “Fairy Shrimp Lengths, Survival vs. TDS for Artemia Urmiana in Iran” and “Fairy Shrimp Lengths, Survival vs. TDS for Artemia Parthenogenetica in Iran”).
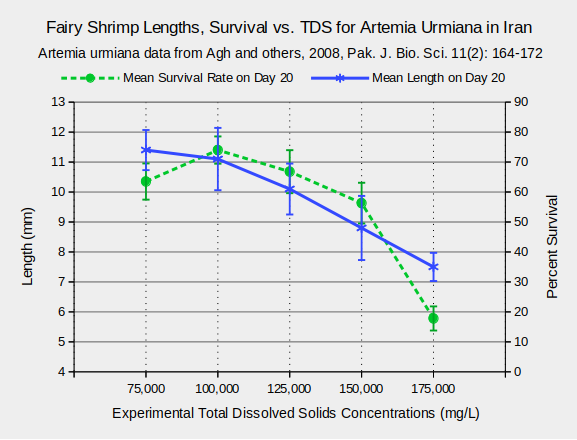
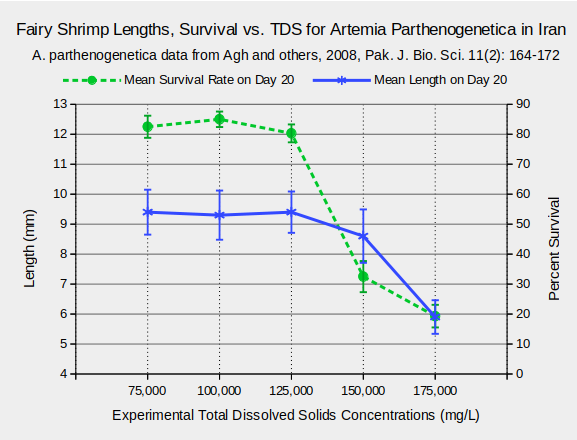
Habitats of Fairy Shrimp – top
5 populations of A. franciscana in 5 different lakes in Mexico did better at TDS concentrations of 100,000 mg/L and 120,000 mg/L than at 60,000 mg/L and 80,000 mg/L (Castro-Mejia and others, 2011). Although there were minor differences between the lake populations, the trends were the same. The graph “Fairy Shrimp Lengths and Survival Rates vs. TDS for Eggs Collected from 5 Lakes in Mexico” shows that survival rates were about 80% after 21 days at concentrations of 100,000 mg/L and 120,000 mg/L, about 65% at 80,000 mg/L, and less than 50% at 60,000 mg/L. Notably, all experimental animals died within 7 days at TDS of 40,000 mg/L (Castro-Mejia and others, 2011). Growth rates, as measured by body lengths at 21 days, increased with TDS concentrations from 60,000 mg/L, to 100,000 mg/L and 120,000 mg/L but those at 80,000 mg/L were oddly less than those at 60,000 mg/L. Irrespective of any intent to find an optimum TDS concentration for raising Artemia franciscana, the results demonstrate that the species is viable in waters with TDS concentrations that differ by a factor of 2.
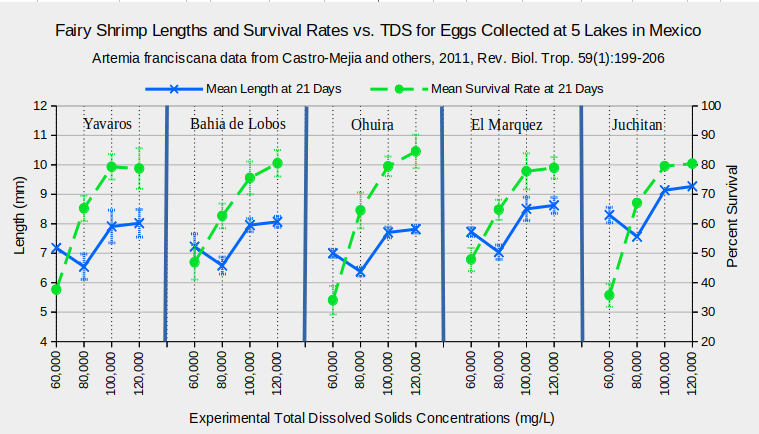
The Artemia sinica population in “Yuncheng Salt Lake”, China, seems to tolerate an even wider range of TDS concentrations. Individuals generally had life spans longer than 40 days whether they were raised in concentrations of 50,000, 100,000, 150,000, or 200,000 mg/L TDS (Yang and Sun, 2023). The graph “Fairy Shrimp Sexual Maturity and Life Span vs. Hours of Light and TDS for Eggs from “Yuncheng Lake”, China” shows a slight decrease in mean lifespan from the lowest to the highest TDS concentration but the 1-standard deviation error bars are quite wide. The time to sexual maturity was longest at 200,000 mg/L and shortest at 100,000 mg/L. The experiments of Yang and Sun (2023) also used different lighting schemes but there do not seem to be consistent differences in lighting effects at different TDS concentrations. The results shown are for experimental temperatures of 25 C. Other trials were at 16 C or 30 C.
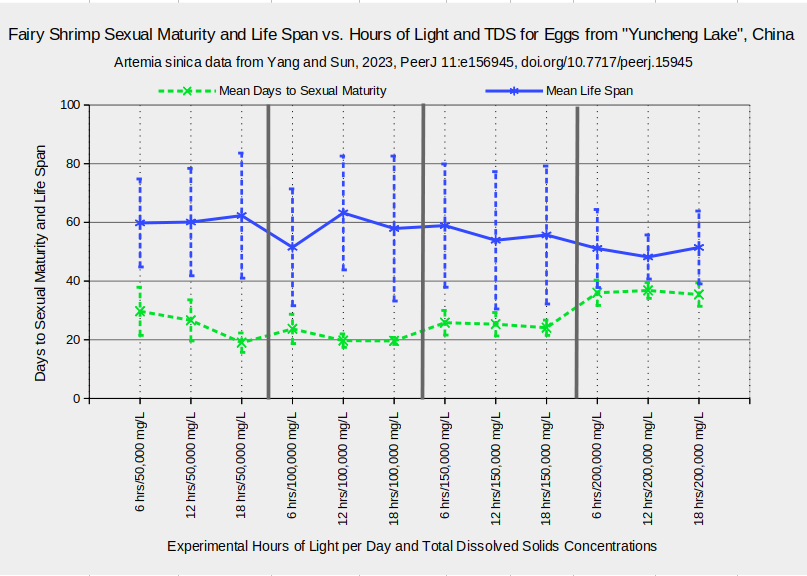
Results for the trials at 25 C.
Equations describing the increase in adult length and increase in the amount of time needed to reach sexual maturity with increasing TDS have been determined for A. monica in “Mono Lake”, California. Lengths are given by a linear equation with a coefficient of determination (r-squared) of 0.89 and days to maturity by an exponential equation with a coefficient of determination of 0.84 (equations in Table J-1 of Jones & Stokes Associates, 1993b). Plugging in TDS concentrations of 60,000 mg/L, 100,000 mg/L (close to the actual lake TDS), and 140,000 mg/L yields lengths of 10.9 mm, 9.5 mm, and 8.1 mm and 35, 45, and 57 days.
Habitats of Fairy Shrimp – top
Egg production is also affected by TDS concentration. Females of the species Artemia urmiana and A. parthenogenetica produce fewer eggs during their lifetimes at TDS concentrations above 100,000 mg/L (Agh and others, 2008). This is due primarily to fewer clutches at higher TDS rather than due to smaller clutch sizes, as shown in the graphs “Fairy Shrimp Egg Production vs. TDS for Artemia urmiana in Iran” and “Fairy Shrimp Egg Production vs. TDS for Artemia parthenogenetica in Iran”.
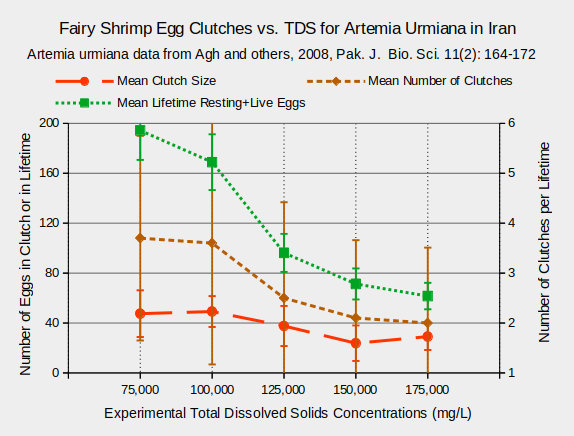
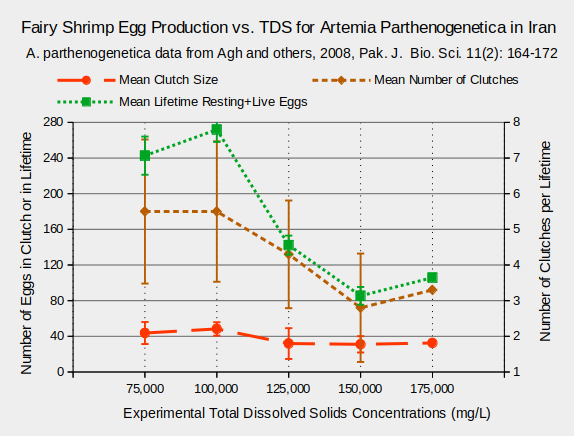
Mean clutch sizes and mean lifetime egg production of Artemia sinica females from “Yuncheng Salt Lake” also decrease as TDS concentrations increase (Yang and Sun, 2023). The graph “Fairy Shrimp Egg Production vs. Hours of Light and TDS for Eggs from “Yuncheng Lake”, China” illustrates the decline. As shown by the very wide 1-standard deviation error bars, the variation of egg production among individuals is huge. For the trial at 18 hours of light per day and 100,000 mg/L TDS, the full range of lifetime egg production is from 4 eggs to 2,698 eggs (the standard deviation is 527 eggs). And this is for fairy shrimp from the same population raised under exactly the same laboratory conditions.
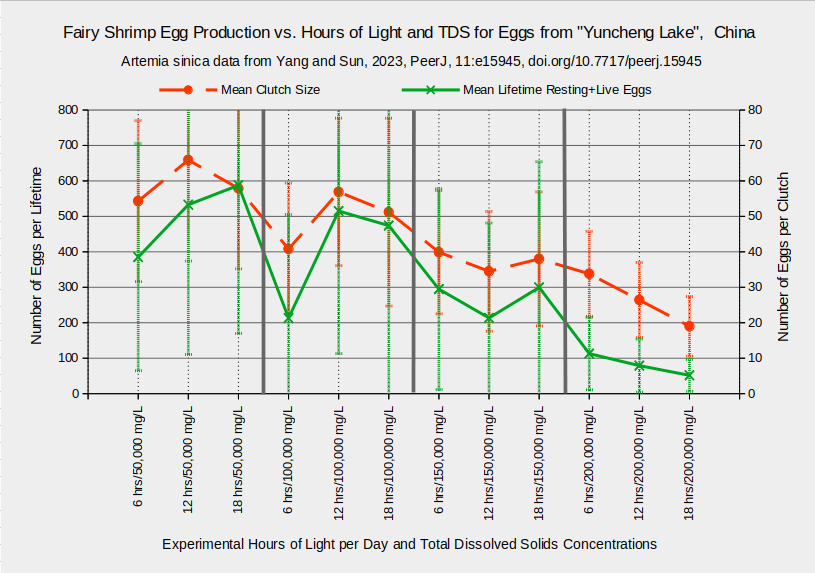
Results are for the trials at 25 C.
The effects TDS on egg production have also been determined for A. monica in “Mono Lake”. Linear regression of the data indicates that the mean number of eggs in clutches after the first clutch are 80, 62, and 45 for TDS concentrations of 60,000 mg/L, 100,000 mg/L (close to the actual lake TDS), and 140,000 mg/L (equation in Table J-1 of Jones & Stokes Associates, 1993b). The coefficient of determination is 0.61. The decrease of clutch size with increasing TDS is similar to that of A. sinica.
Habitats of Fairy Shrimp – top
Switching from experiments to real life, Artemia parthenogenetica populations in 27 Siberian lakes have greater biomass at greater TDS(Litvinenko and others, 2007). The graph “Fairy Shrimp Biomass vs. Total Dissolved Solids of 27 Siberian Lakes” shows a general increase in Artemia biomass from the lowest concentrations of about 51,000 mg/L up to about 200,000 mg/L. The relatively low biomass in lake Ebejty suggests its TDS of 265,000 mg/L has a negative effect on A. parthenogenetica. Very high biomass concentrations in lakes Nevidim and Soljoniy Kulat, which have TDS close to 90,000 mg/L, indicate those lakes have unusual characteristics which promote high population densities that may be unrelated to water chemistry. Excluding Ebejty, Nevidim, and Soljoniy Kulat results in a linear relationship between maximum biomass and TDS that explains over half of the variation in maximum biomass (i.e., r squared greater than 0.5). Mean biomass, which is the mean of approximately 4 monthly samples, also increases with TDS but the data are more scattered. In contrast to laboratory experiments, these results indicate that A. parthenogenetica in Siberia not only can but actually do live in waters with a wide range of TDS concentrations.
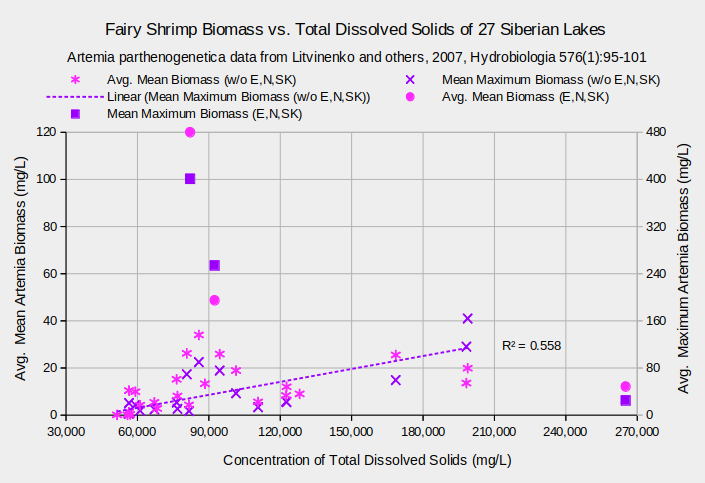
Biological means and averages are of monthly April through October samples of eggs from plankton or bottom samplers or of 60-100 adults from each lake.
Concentrations are means of approximately 4 water samples per lake.
Data for lakes Ebejty, Nevidim, and Soljoniy Kulat are plotted in a series separate from the other 24 lakes.
As shown above (graph “Fairy Shrimp Lengths, Survival vs. TDS for Artemia Parthenogenetica in Iran”), the survival rates of A. parthenogenetica in Iran diminished at TDS concentrations above 100,000 mg/L. One would expect a similar decrease in biomass for that experiment. That different populations of A. parthenogenetica in different environments may respond differently to TDS is not too surprising. Other factors may be involved. Temperature is an obvious difference between Siberia and Iran.
Litvinenko and others (2007) offered a non-physiological explanation for the increase in biomass of Siberian A. parthenogenetica with increase in TDS. Other zooplankton, such as cladocerans, copepods and rotifers, were more abundant in lakes with lower TDS concentrations, particularly those with less than about 76,000 mg/L. Food competition could have limited the Artemia populations in those lakes. In lakes with TDS greater than 167,000 mg/L, A. parthenogenetica was essentially the only zooplankton species.
The relation between maximum Artemia biomass and TDS looks strong. The correlation coefficient, r, for maximum biomass and TDS would have a confidence level of better than 99.9% if that were the only coefficient I calculated. However, I determined correlations of biomass with 8 hydrochemical variables and that increases the chances of a false conclusion by a factor of 8. The 99.9% confidence is no longer attainable but the critical value of 0.697 for the 99% confidence level is still below the correlation coefficient for maximum biomass and TDS. But then I repeated the calculations for 5 biological variables other than biomass. That knocks the confidence level down again. Nonetheless, the correlation coefficient of 0.747 is reliable at the 95% confidence level.
Although TDS affects A. parthenogenetica biomass in the Siberian lakes, it doesn’t affect other life characteristics. Lengths of adult females range between about 9 and 12 mm across all TDS concentrations (graph “Fairy Shrimp Lengths and Clutch Sizes vs. Total Dissolved Solids of 27 Siberian Lakes”). On the same graph, clutch sizes scatter from 7 to 46 eggs for the common TDS concentrations less than about 130,000 mg/L. The 4 results at higher concentrations are within this range and too few to establish a trend. A graph of the numbers of floating and bottom eggs versus TDS concentrations, “Fairy Shrimp Egg Production vs. Total Dissolved Solids of 27 Siberian Lakes”, is a wedge-shaped scattergram with no consistent trends. Graphs of these life characteristics against the individual solutes as weight percents are similarly trendless.
Habitats of Fairy Shrimp – top
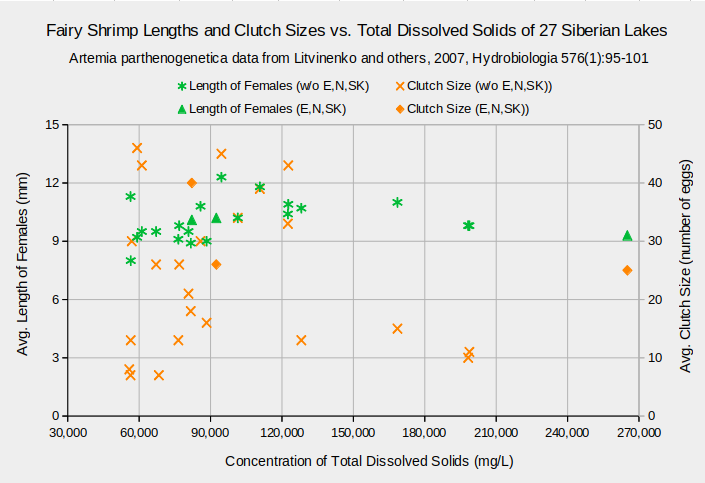
Biological means and averages are of monthly April through October samples of eggs from plankton or bottom samplers or of 60-100 adults from each lake.
Concentrations are means of approximately 4 water samples per lake.
Data for lakes Ebejty, Nevidim, and Soljoniy Kulat are plotted in a series separate from the other 24 lakes.
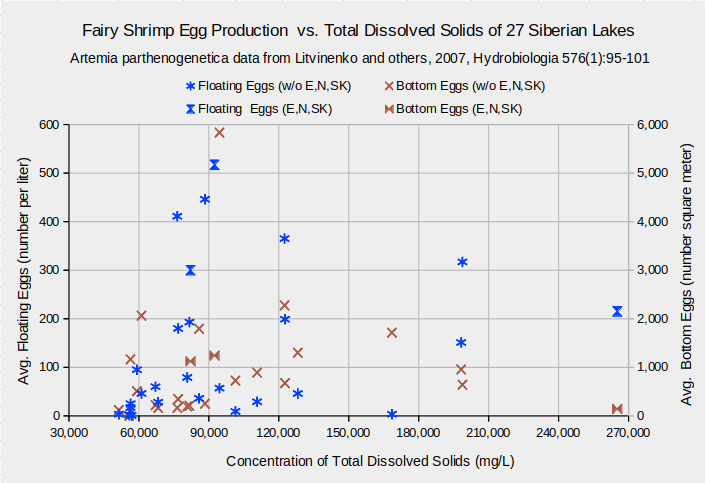
Biological means and averages are of monthly April through October samples of eggs from plankton or bottom samplers or of 60-100 adults from each lake.
Concentrations are means of approximately 4 water samples per lake.
Data for lakes Ebejty, Nevidim, and Soljoniy Kulat are plotted in a series separate from the other 24 lakes.
TDS is a measure of all the solutes dissolved in a body of water. Looking only at TDS ignores the possibility that different solutes have different biological effects. That’s fine for ocean water but individual solute concentrations in ponds and lakes can vary by orders of magnitude. Potassium, magnesium and calcium concentrations affected the survival of pre-adult Artemia franciscana in experimental tests (Bowen and others, 1988, abstract only). Survival rates differed between the different populations of A. franciscana that were tested.
Few studies report concentrations of all the major ions but the study of 27 Siberian lakes with Artemia parthenogenetica populations reported concentrations of calcium, magnesium, the sum of sodium and potassium (calculated to balance the total negative charges of the anions), chloride, sulfate, bicarbonate, and carbonate (Litvinenko and others, 2007). After adjusting for the reduction in confidence due to calculating correlation coefficients for multiple biological and chemical variables, the coefficients of 0.727 for maximum biomass and chloride, and 0.720 for maximum biomass and sodium + potassium are significant at the 95% confidence level. The linear regression of maximum biomass and sodium + potassium concentration as shown the graph “Fairy Shrimp Biomass vs. Sodium + Potassium Concentrations of 27 Siberian Lakes” is almost as good as that of maximum biomass and TDS, shown above.
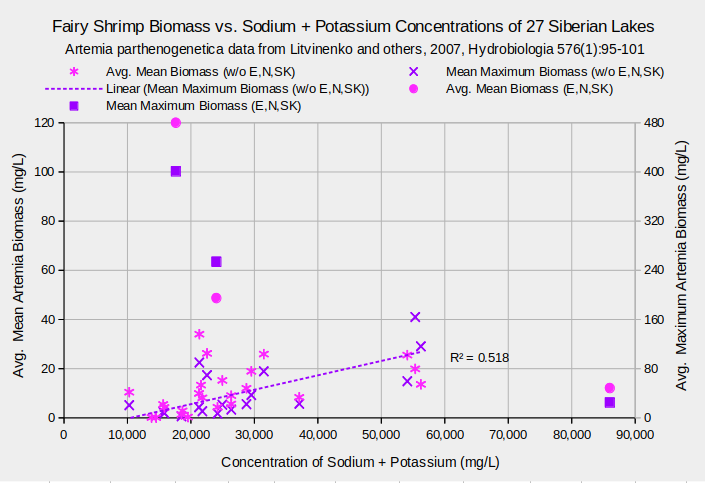
Biological means and averages are of monthly April through October samples of eggs from plankton or bottom samplers or of 60-100 adults from each lake.
Concentrations are means of approximately 4 water samples per lake.
Data for lakes Ebejty, Nevidim, and Soljoniy Kulat are plotted in a series separate from the other 24 lakes.
Habitats of Fairy Shrimp – top
But there is a problem. Sodium + potassium accounts for 18-36% of the dissolved solids in the Siberian lakes. The good correlation of sodium + potassium with Artemia biomass could simply be due to the good correlation of TDS with biomass. In fact, chloride, sodium + potassium, and sulfate make up the largest percentages of TDS, in that order, and have respective correlation coefficients of 0.964, 0.953, and 0.670 with TDS. When the concentrations of chloride, sodium + potassium, and sulfate are divided by TDS to convert mg/L to weight percent of total dissolved solids the correlation coefficients drop to less than 0.10. The sulfate correlation turns negative but not significantly so. That shouldn’t happen if they have consistent and meaningful effects on the A. parthenogenetica populations. The graph “Fairy Shrimp Biomass vs. Sodium + Potassium Weight Percents of 27 Siberian Lakes” illustrates that the linear regression of maximum biomass with weight percent sodium + potassium is a flat line with a vanishingly small coefficient of determination. The graphs of biomass versus chloride, sulfate, magnesium, calcium, bicarbonate, and carbonate weight percents similarly show negligible correlations. It appears that the biomass of Siberian A. parthenogenetica is not much affected by concentrations of individual major solutes. The effects seen by Bowen and others (1988, abstract only) may only occur at concentrations outside the ranges experienced by populations in their natural habitats.
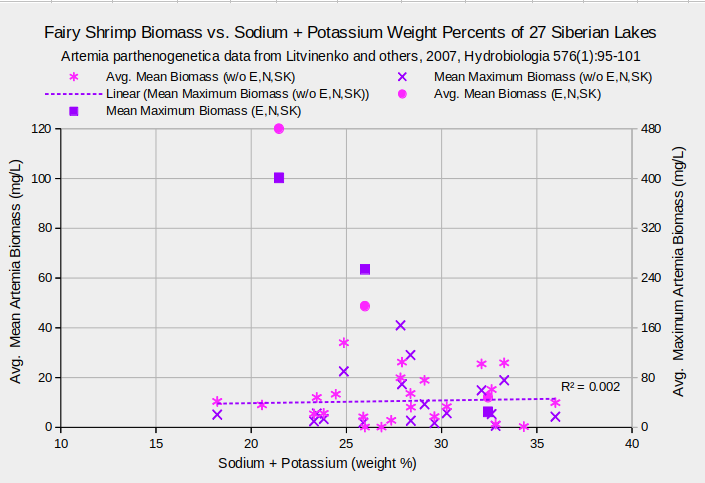
Biological means and averages are of monthly April through October samples of eggs from plankton or bottom samplers or of 60-100 adults from each lake.
Concentrations are means of approximately 4 water samples per lake.
Data for lakes Ebejty, Nevidim, and Soljoniy Kulat are plotted in a series separate from the other 24 lakes.
Different concentrations of major solutes also had no effect on hatching rates of Streptocephalus torvicornis. Hatching rates were approximately the same regardless of whether the only cation was sodium or potassium and of whether the only anion was chloride or nitrate (Beladjal and others, 2007b).
Habitats of Fairy Shrimp – top
Turbidity of Fairy Shrimp Habitats
In a very helpful table, Eng, Belk, and Eriksen (1990) reported turbidities by species for several of the anostracan species in California. They listed means, standard deviations, and ranges for each species. The range from the least turbid to the most turbid measurement obtained regardless of species is 3 to 15,800 (NTU or JTU). In practical terms, that means from very clear to very opaque. The most turbid waters were found in the playa lakes inhabited by Branchinecta mackini, Branchinecta gigas, and Branchinecta lindahli. Suspended clay from the floors of the playas causes the turbidity. Fairy shrimp have struck it rich by adapting to turbid waters as they are likely to have fewer predators and fewer competitors than in clearer waters. Although predatory fairy shrimp inhabit such waters, they can’t extirpate the fairy shrimp prey species without extirpating themselves. The downside of turbidity is that the negligible penetration of sunlight inhibits photosynthesis by phytoplankton. The fact that population densities in opaque water can be high (e.g., videos of Bouncing Acrobats of the Bodie Hills on the Fairy Shrimp Videos page) indicate that fairy shrimp have found some food(s) to compensate for the paucity of phytoplankton.
What does the above discussion on the physical and chemical conditions of fairy shrimp habitats mean to the fairy shrimper? Unless some sort of acid pollution or weathering of sulfide minerals has lowered the pH below 5, some species of fairy shrimp can probably live in the water as long as the biological conditions are permissible. If you want to find fairy shrimp, just get out there and have a look.
Habitats of Fairy Shrimp – top
Fairy Shrimp Osmoregulation and Thermoregulation
Fairy shrimp live in waters with wide ranges of TDS and individual fairy shrimp may experience wide changes in TDS over their lifetimes. To maintain the integrity of the intracellular and intercellular fluids, the animals regulate the exchange of ions and organic molecules with the water they live in. This process of osmoregulation is as important for survival in fairy shrimp as it is in other species which experience large changes in TDS, such as crustaceans that live in estuaries and salmon which live in both marine and fresh water. Consequently, it has attracted some research interest. The only question answered in the papers I have read is whether fairy shrimp maintain the TDS (in some cases only sodium and chloride ions have been measured in experiments) of their intercellular fluids (also referred to as hemolymph) above or below the TDS of the water they live in. The ramifications of different osmoregulation strategies has not been discussed but the fact that fairy shrimp manage it over a huge range of TDS is worth noting.
Fairy shrimp of the genus Artemia live in high-TDS water, well above the TDS of seawater. Permeable cells exposed to such water would dehydrate as water flows toward the higher ionic concentration. Conversely, if not blocked, dissolved ions would flow toward the lower concentration into the cell. To help prevent that, the external surfaces of Artemia, and presumably other genera, have low permeability except for the parts of the legs used for breathing, the epipodites (Kaestner, 1970). The intercellular fluid is nonetheless exposed to high TDS water through the gut. With high-TDS water, this results in sodium and chloride flowing into the intercellular fluid. To counteract that, fairy shrimp excrete sodium and chloride through the epipodites (Kaestner, 1970, p. 92). This keeps ionic concentrations in the intercellular fluid below those of the surrounding water. The important role of the epipodites was cleverly proven by destroying them with immersion in a potassium permanganate solution and finding that the internal and external osmotic pressures in the fairy shrimp bodies became equal (Kaestner, 1970, Table 4-1, p. 93).
Artemia populations are not found in waters with TDS concentrations less than that of seawater but that doesn’t stop experiments at low TDS. The TDS concentration of the intercellular fluid in Artemia switches to being greater than that of the external water when the concentration of the water is reduced to about 9,000 mg/L (“1/4 seawater”, Kaestner, 1970, p. 93). This presumably disrupts critical regulatory systems because Artemia does not survive long at such low concentrations. “Artemia dies within 24 hr” in “freshwater”, which Kaestner (1970, p. 93) did not define.
Recognizing that Branchinecta campestris sometimes occurs with Artemia and also survives in high-TDS water, Broch (1969) measured their intercellular fluid compared to water at various concentrations. At moderate external TDS concentrations, intercellular fluid concentrations were higher. This would allow water to flow into the intercellular fluid. At high external TDS concentrations, intercellular fluid concentrations were nearly the same. At the highest external TDS concentration tested, all the animals died within 24 hours.
Similarly, Branchinecta sandiegonensis and Streptocephalus woottoni maintain relatively constant internal sodium concentrations of approximately 1,886 mg/L and 1,587 mg/L, respectively, over a range of water sodium concentrations of 12-1,380 mg/L (Gonzalez and others, 1996). However, at a water concentration of 1,840 mg/L sodium, both species increased the sodium concentration of the intercellular fluid to about 2,415 mg/L (Gonzalez, 1996). This no doubt stresses the animals’ osmoregulatory systems. In water with 2,300 mg/L sodium, over half of Branchinecta sandiegonensis and all of the Streptocephalus woottoni individuals died.
Branchinecta mackini and Branchinecta lindahli were somewhat hardier in the experiments by Gonzalez and others (1996). Instead of maintaining a near-constant sodium concentration in the intercellular fluid, they both allowed it to increase as the concentration in the water increased, but at a slower rate. At water sodium concentrations of 12 mg/L, intercellular fluid concentrations were about 1,240 mg/L higher. At water sodium concentrations of 1,840 mg/L, intercellular fluid concentrations were only about 350 mg/L higher and in water with 2,300 mg/L sodium they were almost the same. None of these test animals died at 2,300 mg/L.
In addition to TDS changes, fairy shrimp may also experience dramatic changes in temperature when they live in shallow water. Fairy shrimp, like other crustaceans, do not regulate their body temperature. Nonetheless, their cells somehow work at 5 C as well as at 30 C, depending on species. Individual fairy shrimp in the ponds in “Rabbit Dry” Lake in the Mojave Desert experienced changes of up to 19 C in a single day (Brown and Carpelan, 1971). Coincidentally, a pond with fairy shrimp in Antarctica also had a maximum daily temperature range of 19 C (Peck, 2004). A human whose internal body temperature increases more than 6 C above normal usually dies (en.wikipedia.org/wiki/Human_body_temperature). It makes you wonder what’s wrong with warm-blooded animals.
Belk (1977) tested the changes of temperature that fairy shrimp could tolerate. Female Streptocephalus mackini were acclimated to 11.5 C and 25 C water for 24 hours. When transferred to 39 C water, all of the low temperature group died but only 3% of the high temperature group did.
Habitats of Fairy Shrimp – top