The system used by fairy shrimp to collect and process food may seem utterly alien to people habituated to choosing their foods, although it is not uncommon in the animal world. Food particles are strained from the water by bristles, or setae, on the legs. The fairy shrimp diet consists of whatever is floating in the water.
The leg motion that captures food is not the leg motion that propels a fairy shrimp through the water. I have seen fairy shrimp moving their legs vigorously while moving through the water very little or not at all, particularly those living in opaque water. For examples, see Macari East Fairy Shrimp Video 2022-01-31a-r under Clay Dancers of “Carson Lake” Playa or the opening scene of Playa Wire Gate Pond Fairy Shrimp Video 2022-02-10b-r under Digging in the Shallows of Soda Spring Valley on the Fairy Shrimp Videos page. The clay suspended in the water makes it clear in these videos that the water passed through the legs for feeding moves from the front of the fairy shrimp to the rear. Coarse material is brushed aside by the legs and expelled toward the rear. The food, however, is caught between pairs of legs on each side of the body and is then somehow manipulated by the legs to move toward the fairy shrimp’s body and into its food groove. The food groove is a wrinkle that extends the length of the thorax between the left and right sets of legs. Because fairy shrimp swim with their backs down, the food groove is open toward the surface of the water. Food is passed toward the head up the groove by lobes or bumps on the lower parts of the legs according to Pennak (1978, p, 330) but he didn’t explain how.
How fairy shrimp use their legs to strain and push food forward toward the mouth while pushing water backwards to swim would seem a remarkable accomplishment. Kaestner (1970, p. 91-92) provided a diagram and described the process in some detail but it is nonetheless hard to grasp. It involves suction. Walossek (1993, p. 60-61) also diagrammed and described the process which he inferred to occur in fossil Rehbachiella. His Figure 38 offers an understandable explanation. Looking down, the legs are convex toward the front of the animal and bristles extend from the inner ends of the legs over the food groove and point toward the rear. When the outer edge of the leg moves toward the rear to push water back, the bristles on the inner edge move forward in a levered or rotational motion. If bristles extended into the food groove, they could physically push food forward but they apparently don’t. Instead, just as water is pulled into the space forward of a paddle being pushed backward, water and food in the food groove could be pulled forward by the leg lobes and bristles. Walossek (1993) explained it differently though. In his view, water is pulled from the inner edges of the legs through the spaces between the legs and is expelled between the outer edges of the legs during a “stroke” and this action pulls the food forward.
When the food in the food groove reaches the head, it passes over 2 pairs of maxillae and a pair of mandibles before entering the mouth (Dexter, 1959, p. 559). The mandibles are large, relative to body size, and vertical but the grooved grinding surfaces are bent horizontally over the food groove (Belk, 1982). A finger-shaped labrum serves as a lip over the food groove next to the mouth (Linder, 1941). Mastication is accomplished by grinding between the mandibles outside of the mouth. The degree of mastication varies by species. The fecal pellets of Branchipus schaefferi are an amorphous mass whereas those of Streptocepahlus torvicornis have many intact cells (Beladjal and others, 1997). Larger particles or excess food is sloughed off (Kaestner, 1970, p. 92; Pennak, 1978, p. 330). Mucus is added to the food under the labrum before entering the mouth (Kaestner, 1970, p. 92). From the mouth, food enters a short fore-gut, or esophagus, and proceeds through a globular stomach before leaving the head and entering the intestine. The intestine is a narrow tube that extends all the way through the thorax and abdomen to the anus (Dexter, 1959, 559; Belk, 1982). You may occasionally see a string of food material that has left the anus but hasn’t yet dispersed in the water due to the stickiness of the intestine contents.
Fairy shrimp are said to be non-selective filter-feeders. When placed in a suspended mixture of phytoplankton and silt, fairy shrimp of the species Artemia franciscana from “Great Salt Lake” ingested approximately equal amounts of each (Reeve, 1963b). Although Reeve (1963b) referred to the inorganic particles as sand, their diameters ranged from 3 to 40 micrometers with a mean of 7 micrometers (Reeve’s 1963b notation used only the Greek letter mu, rather than mu followed by “m” for micrometer). On the Wentworth grain size scale, silt has sizes of 4-62 micrometers (0.004-0.062 mm or 1/256-1/16 mm). The suspended constituents “were present in roughly equal proportions” and were kept in suspension by continuous, slow rotation of the jars containing the A. franciscana (Reeve (1963b). Moreover, the average volumes of silt and phytoplankton particles were similar at 180 cubic micrometers and 113 cubic micrometers, respectively. The feeding experiments lasted for 14-20 hours.
Additional silt-phytoplankton experiments showed that the Artemia compensated for the lack of nutrition in the ingested silt by eating more of the suspended mixture. Reeve (1963b) varied the proportions of silt and phytoplankton while keeping the total volume of the suspended mixture the same. The ingestion rate increased almost linearly (my visual estimate of graphed data) for the 4 lowest silt concentrations but then dropped off for the 3 highest. Ingestion continued even with 100% silt. Reeve (1963b) also noted that more fecal pellets were produced at higher silt concentrations because the silt does not lose mass by digestion and all of it must be excreted.
In an even more dramatic demonstration of fairy shrimp eating suspended dirt, Beladjal and others (1997) conducted experiments with 2 species of fairy shrimp using colored chalk or clay and algae. When starved fairy shrimp were placed in suspensions of colored chalk or clay with the colors being changed every 10 minutes, each color was seen to move through the digestive systems of the fairy shrimp in order without any intermixing. 65-85% (my visual estimates from graphs) of the initially empty digestive systems were filled in the first 10 minutes and it usually took 30-40 minutes for the leading edge of the colored material to reach the anus. Significant compaction of the chalk occurred as colored strings near the anus were down to 40% the length of those near the head (Beladjal and others, 1997). It is hard to imagine particles as small as clay (less than 0.004 mm) being strained from the water and passed up the food groove by fairy shrimp but Beladjal and others (1997) did not report particle sizes.
When suspensions of algae were alternated with suspensions of chalk or clay, the lengths of algae strings in the guts were shorter than those of chalk or clay and it took longer for the algae strings to reach the anus than for the previous string of chalk or clay. These effects were stronger for Branchipus schaefferi than for Streptocephalus torvicornis (Beladjal and others, 1997). This is likely due to a greater degree of digestion by B. schaefferi. The algae in fecal pellets of B. schaefferi were reduced to an “amorphous greenish-yellow mass” while numerous intact algae cells were observed in the fecal pellets of S. torvicornis (Beladjal and others, 1997). Although B. schaefferi and S. torvicornis sometimes occur in the same ponds and hatch at the same times, B. schaefferi grows faster and dies sooner than S. torvicornis (Beladjal and others, 2003).
While being non-selective, fairy shrimp adjust their ingestion rates depending on the sizes and concentrations of food particles in their environments. In yet more feeding experiments, Reeve (1963a) found that ingestion rates increased up to a maximum as food concentrations increased from 2 to more than 100 food cells per cubic millimeter and maximum ingestion rates were higher for smaller food cells. The experiments again used Artemia franciscana and the 2 food types were approximately spherical phytoplankton species with mean cell diameters of 3.9 and 8.7 micrometers. The maximum ingestion rate of the smaller sized species was about 10 times higher than that of the larger sized species while its cell volume was about 10 times less. Consequently, the total volumes and masses ingested over time were about the same for both food sizes. Controlling ingestion rate is critical. If fairy shrimp did not cap their ingestion rates when food is abundant, then the food particles could end up traveling through the digestive system so fast that the food couldn’t be digested (Reeve, 1963a).
Unlike filter-feeders which can stop feeding when food concentrations are high (Reeve, 1963a, gave examples), fairy shrimp apparently never stop filtering and ingesting. In experiments with a non-spherical phytoplankton species at high food concentrations, Reeve (1963a) observed that food cells accumulated against the labrum above the mouth and piled up until a forward movement of the first pair of legs smashed the ball of food and the back stroke dispersed the cells into the water. At even higher concentrations, blobs of food collected on the food groove and hindered leg movement to the point that the rate of the back and forth leg motion decreased. This suggests that fairy shrimp may suffer ill effects at dense concentrations of food, such as may be present in ponds that have become eutrophic due to fertilizer- or livestock-polluted run-off. Maybe too much algae is what killed off the fairy shrimp in a puddle near Smith Creek US 50 Well Pond.
Fairy shrimp have another mode of feeding, which is less discussed: scraping. They use the bristles on their legs “to scrape food items from surfaces” (Belk 1982). Johansen (1921, p. 23) often observed arctic fairy shrimp ” ‘browsing’ in the mud-bottom of the pond or among the green algae there”. That the arctic fairy shrimp were really eating something on the bottom of the pond rather than exercising or just fidgeting was later confirmed by the identification of 4-keto-myxoxanthophyll in Branchinecta paludosa. This pigment could only have come from cyanobacteria in the mats on the bottoms of many arctic ponds (Rautio and others, 2009). Branchinecta gaini routinely scrape chunks of moss and algae off pond bottoms in Antarctica (Hawes, 2008). In the laboratory, they were observed to swim with chunks of moss held by their legs and to ultimately break them apart with leg motions into edible bits (Hawes, 2008).
As I have observed scraping behavior numerous times in at least 3 different species, I suspect it is not unusual. It is obviously not filter-feeding and it is obviously selective because a fairy shrimp chooses where to scrape. How important scraping is probably varies by habitat and species.
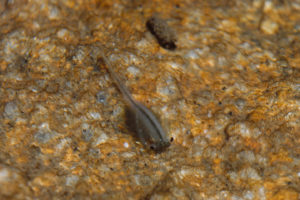
A male fairy shrimp scraping rock in Bivouac Lake, Wind River Mountains. The rock looks bare but maybe it has some cyanobacteria.
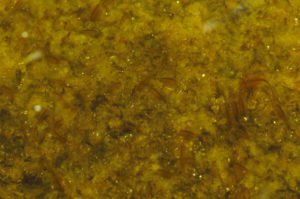
Almost all these fairy shrimp in Dead Ant Rock Pool, Granite Mountains, are on the bottom of the rock pool with their backs up, as indicated by the easily visible, dark intestines that masquerade as backbones. They are not filter-feeding, they are scraping rock for food.
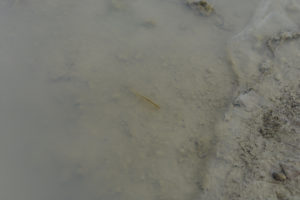
A female fairy shrimp in Bass Flat Southwest Pond, “Carson Lake” Playa with backside up. It is evidently scraping the clayey pond bottom for food. This photograph was taken in early February and there is ice on the surface of the water at upper right.
The video “Garfield 5890 Pond Fairy Shrimp Video 2022-03-14cm-r”, in the section “Back and Forth Life on the Edge of a Pond” on the Fairy Shrimp Videos page, shows a fairy shrimp at lower right aggressively scraping a muddy bottom during the interval 2:36-2:45.
“Playa Wire Gate Pond Fairy Shrimp Video 2022-02-10cc-r”, in the section “Digging in the Shallows of Soda Spring Valley” on the Fairy Shrimp Videos page, shows an extreme example of scraping. Some fairy shrimp have dug themselves into small pits on the muddy pond bottom and die when the pits dry up.
Fairy shrimp eat small things. This is a consequence of the small width of the food groove and the diameter of the mouth. Bristle spacing must somehow control the size of the smallest particle that can be eaten and might also exclude coarse particles. Citing Ussing (1938), Reeve (1963b) mentioned a lower limit of “filtering setule” spacing as 0.006 mm. The small things that fairy shrimp eat include bacteria, algae, protozoans, rotifers, diatoms, and small particles of unspecified organic matter (Moore, 1969, p. R178; Kaestner, 1970, p. 90; Pennak, 1978, p. 330; Belk, 1982). Although branchiopod specialists haven’t mentioned fungal spores as possible food, mycologists have (e.g., Strullu-Derrien and others, 2016).
In one analysis of gut contents, 60 Streptocephalus dichotomus individuals collected from a carp fingerling tank in India (evidently before the carp hatched) were found to have consumed 86% phytoplankton and 14% zooplankton (Selvarani, 2009). About 7.5% of the zooplankton portion consisted of unidentified crustacean nauplii, cladocerans of the genus Moina, Rhinediaptomus indicus copepods, copepod remnants, and anostracan remnants. In decreasing order of abundance, other zooplankton types included eggs (copepod, rotifer, and cladoceran), rotifers, and ciliate protozoans (Selvarani, 2009). 30 immature individuals 6-9 mm long consumed only phytoplankton (Selvarani, 2009), perhaps due to size constraints. Ali and others (1996) found that immature Streptocephalus proboscideus averaging 8.4 mm long consumed rotifers and cladocerans with smaller maximum sizes than those eaten by longer individuals. Phytoplankton was not available in the experiments of Ali and others (1996).
The preponderance of phytoplankton in fairy shrimp diets could be because they are more abundant and smaller than zooplankton. The green algae Chlamydomonas has diameters of 0.009-0.020 mm (as reported by Lukic and others, 2023). Reeve (1963a) measured cell diameters of 0.0039 mm for the green algae Chlorella and 0.0087 mm for Dunaliella. In contrast, half-lengths of 7 rotifer species used in the experiments of Ali and others (1996) were greater than 0.05 mm and up to 0.11 mm and half-lengths of cladocerans were greater than 0.17 mm. In the experiments of Ali and others (1996), the 2 food items eaten in the greatest quantities (my visual estimates of graphed quantities) were the rotifers Keratella cochlearis and Pompholyx sulcata, which were the 2 most abundant of the foods and were also the smallest foods offered, at about 0.105 mm long, or 0.052 mm half-length.
Fairy shrimp may have difficulty ingesting food particles smaller than 0.004 mm in diameter. When offered the very small (diameter 0.002-0.003 mm), spherical algae genus Mychonastes or the larger (0.009-0.020 mm), ellipsoidal algae genus Chlamydomonas in separate trials, Branchinecta orientalis ate more than 5 times more (my visual estimate of the graphed data) of the larger genus over a duration of 1 hour (Lukic and others, 2023). Only 1 food item was offered in each trial.
Fairy shrimp have been referred to as predators (Lukic and others, 2023; Ali and others, 1996). As mentioned above, fairy shrimp eat rotifers, which are microscopic animals. In laboratory feeding experiments with or without additional phytoplankton, fairy shrimp ate copepod nauplii (Crustacea: class Maxillopoda, subclass Copepoda) 0.20-0.32 mm long (Lukic and others, 2023) and the cladoceran Bosmina longirostris (Crustacea: class Branchiopoda, order Anomopoda, family Bosminidae) about 0.35 mm long (Ali and others, 1996). Nonetheless, fairy shrimp are filter-feeders. They do not pursue or ambush prey.
Fairy shrimp have also been referred to as cannibals. Some of the eggs of the species Streptocephalus proboscideus hatch shortly after being released into the pond water rather than developing into resting eggs that remain viable after the pond dries up. The newly hatched nauplii are small, probably less than 0.30 mm long. If the adult population is so abundant that it can filter the entire volume of the pond in about a day (approximately 1 adult per liter of water), then a large proportion of the emerging nauplii are eaten by the adults (Dumont and Ali, 2004, abstract only).
A similar situation could occur in “Great Salt Lake” and in “Mono Lake”. In both lakes, the Artemia franciscana and Artemia monica, respectively, that hatch in the spring produce eggs that hatch before or soon after being released into the water every year. In “Great Salt Lake”, nauplii hatchlings increased in abundance from April to June 1995 to more than 3 individuals per liter, at which time the population of juveniles plus adults reached about 3.5 individuals per liter (Wurtsbaugh and Gliwicz, 2001). In normal years, the density of juvenile and adult A. franciscana is 5-18 individuals per liter in May-June (Wurtsbaugh and Berry, 1990). Densities of 6-8 per liter occur in “Mono Lake” (Wurtsbaugh and Gliwicz, 2001). These reported fairy shrimp densities are high enough to cause cannibalism according to the measure of Dumont and Ali (2004) but I haven’t read of anostracan cannibalism in either lake. Maybe the Artemia species don’t filter water as quickly as the larger S. proboscideus.
In the laboratory, Branchinecta gaini adults from Antarctica were observed and photographed clustering around, dismembering, and consuming a dead fairy shrimp (Hawes, 2008). That could also be considered cannibalism. Lacking an exoskeleton, shell, or carapace, fairy shrimp are soft. Their bodies seem to fall apart within a day or 2 of death (based on samples I was unable to preserve in the field with ethanol). Dismembering them would be relatively easy even with the soft legs of fairy shrimp. Hawes (2008) likened B. gaini’s consumption of dead fairy shrimp to their ability to feed by scraping moss and algae off pond bottoms and breaking them up into small pieces.
3 unusually large fairy shrimp species do not eat just small things. See the “Fairy Shrimp Predators” section on the Predators of Fairy Shrimp page.
The filter-feeding by fairy shrimp is at least partially thwarted by animals that are hard to catch. “Juvenile B[ranchinecta] orientalis fed slightly more efficiently on [the rotifer] B[ranchionus] plicatilis prey than on the faster and somewhat larger calanoid nauplii [(Crustacea: class Maxillopoda, subclass Copepoda, order Calanoida)], implying that other traits than size per se, such as swimming speed, escape response (i.e., jumping movements), and other motility features, may play an important role in fairy shrimp feeding efficiency” (Lukic and others, 2023). The density of copepod nauplii in the experiments was also less than that of rotifers. In each trial, the fairy shrimp were given only 1 food choice and the trials lasted 1 hour. Ali and others (1996) concluded that the lack of copepod nauplii (size about 0.30 mm) and immature copepods (size about 0.75 mm) in the guts of fairy shrimp which had been offered a variety of foods was due to their ability to escape. The similarly sized cladoceran, Bosmina longirostris (class Branchiopoda: order Anomopoda, family Bosminidae) (size about 0.35 mm), was more commonly eaten even though it was less abundant than copepod nauplii. The jumping rotifer, Polyarthra vulgaris, also escaped predation (Ali and others, 1996). However, the rotifer Epiphanes macrourus was also not eaten even though it was just as abundant as Polyarthra vulgaris, was only slightly larger (0.15 mm vs. 0.12 mm), and was evidently less evasive.
There is at least 1 hint that fairy shrimp are not absolutely non-selective eaters. In microcosm experiments to investigate the distribution of the rotifer Hexarthra in rock pools of the southwestern United States, female Branchinecta mackini ate from 280 to more than 600 rotifers per hour but males had no effect on the numbers of rotifers in the microcosms (Starkweather, 2005, abstract only). Unless there is some sexually dimorphic trait in B. mackini that causes females to capture large numbers of rotifers and impedes males from feeding on rotifers, the females or the males must be actively selecting to eat or not to eat Hexarthra rotifers, respectively.
Although fairy shrimp eat small animals from the water around them, they survive quite well without animal nutrition. Examples of what fairy shrimp have been fed from some of the limited number of laboratory experiments I have read about are as follows.
- Artemia franciscana fed algae (genus Ankistrodesmus) and yeast – Forbes and others, 1992.
- Artemia franciscana fed algae Dunaliella salina and D. tertiolecta – Rode and others, 2011.
- Artemia franciscana fed algae Dunaliella tertiolecta – Tapia and others, 2015.
- Artemia parthenogenetica fed algae Tetraselmis chuii – Cespedes and others, 2017.
- Artemia urmiana fed algae Dunaliella tertiolecta and “chemically treated yeast” – Agh and others, 2008.
- Branchinecta sandiegoensis, B. mackini, B. lindahli, Streptocephalus woottoni fed yeast – Gonzalez and others, 1996.
- Branchinecta orientalis fed algae (genus Scenedesmus) – Atashbar and others, 2012.
- Branchinecta lindahli fed brewer’s yeast and fish flake food (which could include ground up Artemia adults!) – Rogers, 2015.
- Branchinecta lindahli fed brewer’s yeast and powdered algal flakes (better choice!) – Rogers, 2019.
- Branchinella thailandensis fed algae (genus Chlorella) – Saengphan and others, 2005.
- Branchipus schaefferi, Streptocephalus torvicornis fed algae (genus Scenedesmus) – Beladjal and others, 1997.
- Branchipus schaefferi, Streptocephalus torvicornis, Chirocephalus diaphanus fed algae (genus Scenedesmus) and hay infusion with bacteria, protozoa, rotifers and other micrometazoa- Beladjal and others, 2007b.
The fish flake food and the hay infusion contain animal matter but the other 9 examples do not. A sizeable proportion of fairy shrimp researchers evidently think fairy shrimp can do fine on a vegetarian diet.
I haven’t seen any estimates of how much food fairy shrimp need to eat in order to survive but some have probably been made. It is evidently not a lot as fairy shrimp typically occur in lakes considered to have low productivity. “Low productivity” is another way to say “low concentration of phytoplankton”. 2 types of low productivity waters where fairy shrimp commonly occur are oligotrophic (oligo=little, trophic=nourishment – New Webster’s Dictionary) and argillotrophic (argos=white, which clay commonly is). Oligotrophic ponds are clear, have low TDS, and commonly have fish. Alpine ponds are examples. Argillotrophic ponds are opaque and commonly have moderate TDS and pH greater than 8. They occur on clayey soils, such as playas. The North American Lake Management Society, which has a name that suggests it knows what it writes about, made a long argument about why this old lake classification scheme is unworkable and misleading (“Defining Trophic State” at www.nalms.org). Nonetheless, if you read of oligotrophic or argillotrophic lakes in the literature, recognize that they indicate potential fairy shrimp habitat.
Microcosm experiments with fairy shrimp and phytoplankton grown from soils collected from interdune pans with algal crusts in the Mojave Desert of southern California showed that phytoplankton abundance was not limiting for this argillotrophic environment (Bostoff and others, 2010). The phytoplankton in these experiments was dominantly cyanobacteria of the genus Microcoleus and the fairy shrimp were Branchinecta mackini and rare Branchinecta gigas. At the end of 30 or 60 days, the microcosm containers were dried and the concentrations of chlorophyll-a, a proxy for phytoplankton, were measured. Whether the microcosm had fairy shrimp or not made no significant difference for the chlorophyll-a concentration (Bostoff and others, 2010). Confounding factors are that other microscopic foods may have been present and the microcosms had more than twice as many clam shrimp (Branchiopoda:order Spinicaudata, Cyzicus setosa) as fairy shrimp.
Although fairy shrimp have evolved to live in ponds without abundant phytoplankton, the staggering abundances of fairy shrimp in some ponds at some times raises the question of whether populations experience famine. Examples of abundance I have seen include the following.
- “Steamboat Lake” 2nd East Pond
- South “Coon Lake” Pond #3
- Beauty Peak East Pond Fairy Shrimp Video 2021-04-30c-f
(Bouncing Acrobats of the Bodie Hills on the Fairy Shrimp Videos page)
Boom-and-bust cladoceran population dynamics provide a possible model for fairy shrimp famines. Populations of the cladoceran genus Daphnia (Branchiopoda: order Anomopoda, family Daphniidae) typically increase rapidly during a spring algal bloom in northern temperate climates, up to 100-fold, but then contract during a clear-water phase after most of the phytoplankton have been eaten (Thorp and Covich, 2001, p. 867). The population collapse is due to diminished food supply as well as predation, which affects different cladoceran species differently depending on size, among other factors (Thorp and Covich, 2001, p. 867-868). This scenario would require a relatively long-lived pond.
The Artemia franciscana population of “Great Salt Lake” at times exhibits a similar famine cycle. It didn’t in June-October in 1994. Chlorophyll-a concentrations, a proxy for phytoplankton, remained low during June-September 1994 but began rising in late October as summer-hatched adults began dying off. 1995 was a different story. Chlorophyll-a was 16.0 micrograms per liter in March 1995, rose to 28.0 micrograms per liter in April, and then dropped to less than 0.5 micrograms per liter in June. A. franciscana hatched in March with 3.4 nauplii per liter measured on March 17 and began consuming phytoplankton. The A. franciscana population peaked at 8.5 individuals (mostly nauplii and juveniles) per liter on June 9 and collapsed to 4.1 individuals per liter on June 19 (mostly juveniles) (Wurtsbaugh and Gliwicz, 2001) coincident with the collapse of chlorophyll-a concentrations. Adults were less affected. A peak density at almost 1 individual per liter in April was followed by a steady decline to maybe 0.33 individuals per liter on June 19 (estimated from graph).
The Artemia monica population in “Mono Lake” experiences more consistent seasonal famine. “Each year during summer, the shrimp population becomes food limited and the abundance of algae probably affects year-to-year changes in shrimp abundance (Jellison and Melack 1992)” (Jones and Stokes Associates, 1993b).
Both “Great Salt Lake” and “Mono Lake” are permanent water bodies. Identifying famine in ephemeral water bodies would be more difficult because TDS, temperature, and water depth change rapidly and could also cause death. As conditions change from year to year, famine in ephemeral ponds may not re-occur regularly. For the fairy shrimper, who cannot see phytoplankton, rotifers, bacteria and other fairy shrimp food, it is probably not possible to determine when a particular fairy shrimp population is affected by famine or at risk of famine. Just marvel that they are there at all.
Competition for food could limit the distribution of fairy shrimp or the populations of fairy shrimp where they do occur. Kaestner (1970, p. 90) suggested that because fairy shrimp often occur in ponds with no other macroinvertebrates, food competition is not common. However, fairy shrimp do occur with other herbivores or omnivores in many cases. Oftentimes, these are other branchiopods, such as tadpole shrimp, clam shrimp, or cladocerans, notwithstanding that these other branchiopods are likely adapted to somewhat different pond niches than fairy shrimp. Maeda-Martinez and others (1997) found that of 134 ponds in northern Mexico and 166 in Arizona, 45% had more than 1 species of fairy shrimp, tadpole shrimp, or clam shrimp. 36% of 117 ponds observed over an 18 year period in northern Yugoslavia had more than 1 species of large branchiopod (Petrov and Petrov, 1999). All of the 3-species and 4-species ponds included fairy shrimp and 92% of the 2-species ponds did. A survey of ponds in the Eastern Cape Province of South Africa found that 87% of the ponds with large branchiopods had more than 1 species (Mabidi and others, 2016). 12 of the 14 ponds with fairy shrimp also had a species of clam shrimp.
Tadpole shrimp and clam shrimp were commonly found with fairy shrimp in the “Burnt Lake” area of Middle Washoe County as well as in Northeastern “Lewiston Lake”.
An abundance of cladocerans (Crustacea: class Branchiopoda, order Anomopoda, family Daphniidae, genus Daphnia and family Moinidae, genus Moina) was found with Branchinecta mackini in North “Scotty Lake” West Pond in the Antelope Hills.
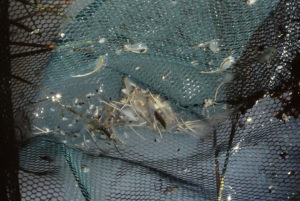
Abundant fairy shrimp and cladocerans caught in one sweep of the net through an argillotrophic pond. The cladocerans are almost transparent, lentil-size blobs with black spots. They are particularly abundant in the left part of the net.
Both cladocerans (Branchiopoda, orders Anomopoda, Ctenopoda, Onychopoda, Haplopoda) and copepods (Crustacea: class Maxillopoda, subclass Copepoda) were found with fairy shrimp in Smith Creek Cold Springs Ponds. In Smith Creek Cold Springs Ponds Fairy Shrimp Video 2021-04-23r (Crowded Cohabitants of Smith Creek Cold Springs Ponds on the Fairy Shrimp Videos page), the cladocerans are considerably smaller than fairy shrimp and the copepods are the even smaller, dark spots.
Food competition is a distinct possibility for some fairy shrimp populations but it has rarely been documented. A microcosm experiment in rock pools in Australia less than 20 cm deep found that microcosms with 5 fairy shrimp (Branchinella longirostris) saw cladoceran (Macrothrix hardingii) numbers decline from 10 to 5 individuals whereas those with 1 or no fairy shrimp saw average declines to 9 cladocerans (Jocque and others, 2010). This was interpreted as evidence of fairy shrimp winning the food competition due greater filter feeding efficiency as a result of their larger sizes. The possibility of predation was not considered.